Flash and JavaScript are required for this feature.
Download the video from Internet Archive.
Description
Professor Martin begins with early mammalian development and the differentiation of cell types, thus setting the stage for reproductive cloning and stem cells.
Instructor: Adam Martin
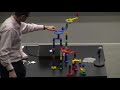
Lecture 35: Reproductive Cl...
ADAM MARTIN: So to start out, I just wanted to mention we talk a lot about dogma in the lab. So we talk about dogma, right? There's the central dogma, which is DNA. Information flows from DNA to RNA to protein.
I'm going to describe another dogma, if you will, which is that life starts as sort of a fertilized egg. So you get a fertilized egg. And this fertilized egg, which you see in the video up here, undergoes development. And as part of that development there is what is known as differentiation, where cells acquire more specialized cell types. So there's development and differentiation.
And this results in adult cell types that are known as differentiated. So it results in differentiated/adult cell types with specialized functions. And you see just a few of them up on the slide above. There are, in fact, thousands of different sort of cell types that humans have.
But the dogma, at least up until fairly recently-- in the past couple of decades or so or at least before 1960, 1950s-- is that this is you unidirectional, that basically the development goes in one direction, where you go from the fertilized egg to the differentiated adult cell types. And so this state down here-- the differentiated adult cell types are fairly stable, right? I mean, if you look at your neighbor you see they don't have muscle growing on the outside of their bodies. Their skin cells stay skin cells. And that is a relatively stable state. OK.
Now, last week you learned about a contradiction to the central dogma, which is the behavior of retroviruses, which have reverse transcriptase, which can sort of go backwards up this pathway, where you can get DNA from RNA. OK. And today, we're going to talk about how you can sort of overcome this you unidirectional path here. And this is going to be what we will call reprogramming.
But first, I want to talk about this differentiation process because it's important for us to understand this before we get into the reprogramming event. And so the fertilized egg is capable of producing all of these different cell types. OK. So the fertilized egg will be known as totipotent, meaning it has the potential to form all cell types.
And then over development, this potency goes down such that when cells differentiate into their final adult form there is a much more limited repertoire of cell types that the cell can go into. And one way of thinking about this is that if you think of this marble run here, the totipotent state is right at the top here, right? If I put a marble in the top here, it has the potential of going into any of these three sort of different fates. OK.
So this is the totipotent state up at the top. And you'll see these marbles will be able to go into any of these three different states here. OK. But you see how that marble went down on this path. Some marbles go down in this path.
And so you can think of cells going through development is sort of getting funneled into these distinct paths. And in this case, it's kind of random. But in development, it relies on signaling between cells and interaction between cells in the multicellular organism.
So as an example, I want to tell you about early mammalian development and the differentiation of cell types in the early mammalian embryo. OK. So we start with an oocyte. That's the female gamete. And the male gamete, the sperm. So these can come together to form the zygote. If I draw a little circle in the middle, it's a nucleus.
And this zygote is totipotent. And the embryo undergoes cleavage divisions, which I'll show here. You see how that zygote divided into two cells. Now it's going to divide into four. And it'll keep dividing till it's 16 probably. So you get cleavage divisions that generate more than one cell.
You start with a single cell. The cleavage divisions give you more than one cell. And what you see up here now is a stage known as the blastocyst or the blastula. You can see there's a hollow sort of inner fluid-filled area here. And you see there are cells around that fluid-filled cavity.
And there's a thickening on one side of the embryo. OK. So I'm going to draw that out here. Sorry. Mine is a little flat up here. It should be perfectly spherical.
And there is this thickening on one side of the blastula, which is a bunch of cells that are kind of interior on the inside of the embryo. These cells on the interior are known as the inner cell mass. And these cells are now restricted in what they can become. These cells will become the embryo proper. So they'll become the cell types that will be a part of the fetus.
These cells on the outside are known as the trophoblast cells. And these cells will form part of the placenta-- the embryonic portion of the placenta. So they form part of the placenta. And they're important for this embryo being able to implant into the uterine wall.
So if we think about-- this is the first example of differentiation in the early mammalian embryo because you can see, based on what I said here, these cells are becoming restricted in what they can become. So this is kind of like the first branch point in differentiation. So we get some more marbles going down here.
All right. So this would be the sort of stage right before the blastula, before differentiation. And you can see these marbles-- when I let them, they will either go this way and become one fate, or they'll go the other way and be sort of directed down another potential fate. There we go.
So this is the first sort of branch point, if you will, in fate determination for the mammalian embryo. And so this branch point here is different from this because once the marble goes one way or the other, it's restricted in what fate it can become.
OK. So these cells here are not totipotent because they can't form the trophoblast. They can't form the part of the placenta needed by the embryo. But they do form the embryo proper. So they are still capable of many fates. And so these cells are known as pluripotent, which means capable of many things
OK. And so a type of cell type, which I'm sure you've heard about, is called Embryonic Stem cells, or ES cells. These ES cells are derived from the inner cell mass. So these would be-- if they were taken out of the blastula-- sorry-- this is called the blastula-- if they are taken from the blastula and cultured in vitro, these would be sort of embryonic stem cells that can be propagated. And they form the embryo proper, so these are pluripotent stem cells that can basically-- they are still capable of forming sort of any of the cell types in the embryo-- capable of forming embryonic cell types.
Now let's look at the next slide. So this branch point-- this first branch point sort of in development is associated with changes in gene expression. So there are changes in gene expression. And you're seeing one up on the slide above. The slide above is a fixed blastula.
And all of the nuclei in the blastula are stained with green. But there is one gene that's stained in red. It's called Oct4. And this is a transcription factor that marks sort of pluripotency. And you can see how it's expressed specifically in these cells of the inner cell mass, which are what the embryonic stem cells are derived from. So there are clearly changes in gene expression.
And one question you might have is whether or not these cells that are going to form the embryo proper, whether they have lost information such that they're unable to form the part of the placenta. And you can also ask this for an adult cell. Has an adult cell in your body-- has it lost gene content such that it's unable to make an entire organism?
I'm going to tell you the answer. I want you to think about what experiment would allow you to sort of determine the answer. But I'm going to tell you the answer is that there is not a loss of gene content. But this differentiation process is due to changes in gene expression for the vast majority of our cell types.
So let's say you wanted to determine whether or not a differentiated cell had lost some sort of capability to regenerate an entire organism. What might be some type of experiment you could do? Brett.
AUDIENCE: Creating conditions that will perhaps be similar to what embryonic stem cells or perhaps [INAUDIBLE] factors that allow for you to change the expression back to more than one impact to see if that would actually change it.
ADAM MARTIN: All right. Great. So Brett had two really good points, I think. The first is to try to sort of reproduce conditions of pluripotency. Or you can even try to reproduce conditions of totipotency, right? In which case you'd want to sort of take the genetic material of the somatic cell and sort of put it back in this situation where it's present sort of in the cytoplasm of the zygote.
And the other point that Brett made is to try to maybe express something that would induce this type of pluripotent state. If we knew exactly what the genes were that create this pluripotent state, maybe we could just express those genes and regenerate sort of a cell that moves from way down here all the way up to the top again.
And, actually, what Brett suggested were the two experiments that won these two folks the Nobel Prize in 2012. So in 2012, the Nobel Prize was awarded jointly to Sir John Gurdon, right here, who incidentally has the best hair of all Nobel laureates, and also Shinya Yamanaka.
And so these two folks were awarded the Nobel Prize for being able to show that mature differentiated cells can be reprogrammed to become pluripotent again, which basically gives us the conclusion that there's not a loss of gene content during the differentiation process. And the work from Yamanaka showed us several genes whose expression is critical to induce this type of reprogramming. And their work spanned from frogs-- so John Gurdon worked on development of frogs, specifically Xenopus laevis, which you've seen before. Shinya Yamanaka's work involved mice and also human cell lines.
So I'm going to tell you about their experiments and how this-- sort of demonstrated this conclusion here. And the first thing I want to show you is an experiment, which is very simple conceptually. Technically it's very complicated, which is if you were able to take a nucleus from an adult cell that's differentiated, could you get it to change by introducing it back into the egg cell or a zygote cell?
So this experiment involves having an oocyte, but in this case an oocyte where the nucleus has been removed. So you take an enucleated oocyte. And want to know if the cytoplasm of this oocyte is somehow special that would allow it to reprogram the nucleus of a differentiated cell.
And so you could suck up the nucleus of a differentiated cell. So a somatic cell is another way to say differentiated. So it's a somatic cell nucleus.
And the experiment conceptually then is to just take this somatic cell nucleus that you've sucked up and inject it into an oocyte without a nucleus and see if the cytoplasm of this oocyte is somehow able to change the properties of the nucleus such that it now is in an undifferentiated state. So you generate oocyte now with the somatic cell nucleus. And the question is whether the somatic cell nucleus is capable of generating the diversity of cell types that are normally present in an organism.
So you could then let this go and develop. You could let it develop into a blastula, which I'm drawing here. So you could generate a blastula. And this will be a way to get embryonic stem cells derived from sort of this type of nucleus.
But you could also let it grow into an entire organism. But this organism would be genetically identical to whatever organism donated this nucleus. So because you're duplicating an organism, that is known as reproductive cloning. So this is reproductive cloning, if you go all the way to the organism.
I'll show you just a little video on the nuclear transfer.
[VIDEO PLAYBACK]
- You see now that this drilling pipe head is going to suck-- drill a little hole into the membrane. You can maybe see a little bit of the hole right here at the next part.
[END PLAYBACK]
ADAM MARTIN: He's talking about this pipette here. The embryo is being held with another pipette over here.
[VIDEO PLAYBACK]
- You can see a bit of the hole. And now the pipette's going to go in and remove the nucleus. And if you look carefully in the pipette, you'll see a line in the nucleus, which are all the chromosomes lined up. So the nucleus is going to be squirted out now because we don't need it anymore. And then we have an enucleated egg.
Now, the next step is to take a set of eggs like that-- and I'll show you two-- and then transfer into them a nucleus from another kind of cell, a fully-differentiated somatic cell. So here, the enucleated egg is set on the side. And it's held by this holding pipette on the left.
There's drilling the little hole in the membrane. Here we go in. Here comes the nucleus from the right.
[END PLAYBACK]
ADAM MARTIN: That's from a somatic zone.
[VIDEO PLAYBACK]
- And these pipettes are operated with a piezoelectric device. So you can't see it here, but it's like a little jackhammer going very quickly, (TRILLING SOUND) like Woody the Woodpecker, getting in there.
[END PLAYBACK]
ADAM MARTIN: I don't know if I would have used Woody the Woodpecker as an analogy, but that's basically the idea. So you can take a nucleus from a somatic cell and transplant it, if you will, into an oocyte and then determine whether you can get either a blastula or an entire organism from that differentiated cell's nucleus.
So this is one result from Sir John Gurdon. And what the experiment was, in this case, was to take oocytes, or eggs, from this wild-type laevis frog and to transplant nuclei from donor frogs that are albino. So you can see this is an elegant experiment in that you can sort of track the origin of the nucleus, because it's genetically marked with this albino sort of phenotype.
So you're getting a nuclei from-- you're getting nuclei from albino tadpoles. So these are differentiated cell nuclei. And they're transplanted into wild-type donor eggs. So normally, the wild-type frog would reproduce frogs that look like it-- that are non-albino.
But in this experiment, Gurdon and his lab were able to get frogs that were albino. So these would be sort of clones of whatever albino tadpoles they got the nuclei from. So you see, in this case, it's the origin of the nucleus that is determining sort of the phenotype of these frogs. So that allowed them to show that the nucleus is getting reprogrammed from this albino tadpole and is able to still generate all of the cell types present in a normal organism. Yes. Brett.
AUDIENCE: So this is an unfertilized egg that they were taking from the--
ADAM MARTIN: Yes.
AUDIENCE: And so they're extracting all this DNA, then putting in a full set of DNA from the albinos.
ADAM MARTIN: Yep.
AUDIENCE: It would go on to differentiate a full set of DNA?
ADAM MARTIN: Yeah.
AUDIENCE: OK.
ADAM MARTIN: Yeah. So, yeah, they're-- and actually, it was taking unfertilized eggs, which is the biggest trick. I think people had tried to do this in frogs before, and it failed because they were using fertilized eggs. And there's something about sort of the oocyte development that makes it better at reprogramming the nucleus.
OK. So that's with frogs. So that experiment was actually done in the late 1950s, published in the early 1960s. And so it took another 40 years or so for the first mammal to be cloned. And you guys probably weren't even born yet.
But for those of us who are older, we remember this because there was a big brouhaha over Dolly the sheep, which was the first cloned mammal. I believe that's Dolly there. So this is-- I'm not sure which one is Dolly. They're both the same type of sheep. So this was done by Ian Wilmut and his group.
One thing I want to point out about this is it's incredibly inefficient. If you look over here, this Dolly resulted from over 400 oocytes having this sort of nuclear transplant take place. So it's not a very efficient process. And so that lack of efficiency is due to the fact that the nucleus is resisting getting reprogrammed.
And this is a graph from one of John Gurdon's sort of-- he wrote a review article on sort of reprogramming after he won the Nobel Prize. This is from that. And what's plotted is sort of the frequency in which transfers results in sort of a differentiated organism. And what's on the x-axis is the stage of the cell that's used to transplant.
And so what you see is that over the course of differentiation it gets harder and harder for the nucleus to get reprogrammed to create sort of all the cell types that are normally present in an adult animal. So nuclei do become more restricted in their ability to be reprogrammed over development. But the fact that any of them are able to be reprogrammed suggests that when they are-- that during differentiation there is not a loss of gene content. And John Gurdon has done a lot to characterize sort of the changes in the nucleus that happen during differentiation which sort of resist this reprogramming by the oocyte cytoplasm.
All right. So mechanistically, what's happening? Well, one big-- one of the people who really showed what's going on there is Shinya Yamanaka. And what his work shows is that you can take just a few genes-- it turns out it is four-- and you can induce reprogramming by just expressing these four genes in an adult differentiated cell. So in this case you have a differentiated cell.
And what he initially used we're fibroblasts, which are a type of differentiated cell. And what Yamanaka showed is that you can express four transcription factors, one of them being this factor here, Oct4, which is expressed in the inner cell mass and was shown to be involved in sort of maintaining pluripotency of these inner cell mass cells. So you could take Oct4 plus another transcription factor, which is important for pluripotency, plus two others and c-Myc. These are the four. So these are all transcription factors.
And what they found is if you express these four transcription factors in a differentiated cell, you could get it to revert to a more pluripotent state. So this results in what is known as Induced Pluripotent Stem cells, or IPS cells, for short.
And then like embryonic stem cells, here these cells can give rise to different cell types. And so one of the goals of this field of developmental biology and cell reprogramming is to use this technology to replace cells that are lost in patients. So this is known as regenerative medicine.
And the idea of the theory of regenerative medicine is to take ES cells or induced pluripotent stem cells from patients and to be able to culture them in vitro-- so culture in vitro-- and then to be able to direct these cultured cells into different cell fates in order to use these cells possibly to transplant them back into an individual where maybe these cell types are dying. So you could then differentiate these cells using certain biochemical signals that you can add to the media in order to create different cell types, like neurons, muscle, maybe skin.
And one example of this was shown by Shinya Yamanaka, where what they did was to take human fibroblast cells, put them back in the pluripotent state by expressing those four factors, and then get these cells to differentiate into cardiac tissue, so cardiac cells.
Here's a movie. So these are from adult fibroblast cells. But now you can see they're beating sort of like a cardiac tissue would. So these were made into IPS stem cells, cultured in vitro, and then directed into a cardiac muscle fate.
So the goal would then to be use these and to transplant them back into a patient, such that if a patient had, let's say, a neurodegenerative disease and was lacking a certain type of neuron, you could then take cells, start them on the path to neuronal development, and then transplant them back into that patient. And if these cells are derived from the DNA of that patient, then there won't be transplant rejection.
Because what regulates transplant rejection is the major histocompatiblity complex locus. And it's polymorphic. But if you're taking a nucleus from the patient and then causing the cells to differentiate in vitro, you're taking the exact same-- you're taking clonal cells and putting them back in the same patient, such that they won't be rejected, ideally.
All right. Now I want to try something with the remainder of our time. I want a group-- all right. Everyone on this side of the room over, I want you over here. And everyone on this side of the room, I want you on this side over here. OK. Maybe you guys can go over here so that we're more balanced.
We're going to have a little debate. You can sit down. It's OK. Be in a position to talk to each other. I want you guys talking to each other. That was the goal of putting you close together. OK.
So in the past couple weeks, there's been a little bit of a stir because there's a researcher in China who has claimed to have made the first gene-edited baby. Perhaps you have heard of this. You probably have because it's been all over the news.
So I want you guys-- let's see. I want us to debate whether we should-- what are the advantages or disadvantages of gene editing or human cloning. You could talk about both. And then I want you guys to be able to present them to me. I'll write them down, and we'll have a discussion about it.
Guys, continue this discussion sort of outside class. I think it's really interesting. And it's going to be something that you're going to hear a lot about in the coming years, I'm sure.