Flash and JavaScript are required for this feature.
Download the video from Internet Archive.
Description
This lecture covers microorganisms and some of the threats they pose to human health, such as infectious diseases. Professor Imperiali also discusses antibiotics and the mechanisms by which bacteria become resistant.
Instructor: Barbara Imperiali
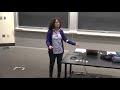
Lecture 32: Infectious Dise...
PROFESSOR: OK. Let's get going here. So this week I'll be talking about bacteria and viruses. And these are really significant topics, because I think it's something that we often don't think about the magnitude of the problems and what kind of crises we're approaching with respect to the therapeutic treatment of infectious disease.
So what I want to try and get home to you this week is the variety of different microorganisms that threaten our health, and just talk to you about the sorts of issues that are really prominent in the news concerning resistance to therapeutic agents. But in order to do that, we've got to meet some bacteria, meet some viruses, and understand that some of their lifestyles, their mechanisms, so that we can understand what kinds of agents are used and developed to try to mitigate these diseases, because it's only through a molecular mechanistic understanding of the life cycles of viruses and bacteria that we can understand how many of these therapeutic agents work and what may be happening in resistance development.
Now I find this particular slide a little daunting, but I want to point out to you that it concerns the world's deadliest animals. So we worry a lot about tigers, and sharks, and things like that, nasty poisonous snakes, bites from dogs with rabies, and so on. I'm going to leave this black bar here, sort of unmentioned. I don't know what year this is, but if we talk about daunting, that's pretty serious. And then the biggest killer on this screen is the mosquito. But it isn't actually the mosquito, it's the protozoal microorganisms that the mosquito carries from one person to another that really make that such a serious consideration.
But what's not here are all the bacteria and viruses that actually are far more serious. And the numbers on the next stage will show you just quite how shocking these numbers are. If you're interested in infectious disease as a field, because I think anyone going towards MD, MD/PhD infectious diseases, it really is a critical area that we have to get to grips with. There are not enough vaccines in the world. There is not enough treatment with a very microbe specific anti-infective agents.
So I encourage you to look at the CDC. There's a few other places where there's loads of information collated, such as the NIAID, which is the NIH Center for Infectious Disease, and the World Health Organization. So there's lots of places where you can find stuff out.
So what we're going to be talking about in the next three classes are our smallest enemies, things like bacteria, fungal infections from things like yeast or Aspergillus, which would cause candida and aspergillosis. Protozoal disease we won't mention, but those are the types of diseases that are carried by things like ticks, mosquitoes, tsetse flies. We think of those as the infectious agent, but it's really what those organisms carry and cause the spread of disease that's important there. And we won't either talk about prion diseases, which are the diseases that don't involve an infectious microorganism, but are believed to be spread from protein to protein through the nucleation of new prions from existing prions.
What we'll focus on in the first class is bacteria and in the other two on viruses, with an eye to looking at antibiotics and antiviral agents, how they work, where they go wrong. And this is where the numbers get fairly shocking. So for example, bacterial infections of the lower respiratory tract, that's deep in the lungs, cause 4 million deaths a year. Think back to the numbers you just saw on that first slide. These are things like strep pneumoniae, Klebsiella pneumoniae. They're called pneumonias because they're infectious diseases of the lung, but the organisms that cause them are of the Streptomyces, and Klebsiella, and Staphylococcus aureus specifically.
But there are others that cause lung infections and lower respiratory disease. These are particularly troublesome in areas where the atmosphere is bad. In big cities where there's a lot of insult from emissions and such that make the lungs weaker, then these sorts of organisms can really take a hold more readily, so they are more serious. There are many, many microorganisms that cause pneumonias. And sometimes it's a real problem to track down the precise microorganism, which makes the issue of treatment really difficult, really challenging. So I'm going to talk in a minute about absolute identification of infectious agents, so we can do better jobs of specifically targeting the causative agents.
Diarrheal disease-- 2 million deaths. These are organisms like Campylobacter jejuni and Salmonella enterica. We tend to have these crises, because romaine my is contaminated with infection. There are very few deaths in the developed world. We get down to that very quickly, say stop eating Romaine lettuce until we figure out what's going on here-- very, very few.
But once again, in the developing world, these can run rampant. And they can grab small children and older people who are already compromised, already a little bit not quite with strong immune systems, and people generally die of dehydration, because these diseases really hit the GI tract. It causes leaking us in the GI tract and really, really serious diarrheal disease. So those are the bad boys there. But once again, there are many others.
Tuberculosis is yet another really serious infectious disease caused by Mycobacterium tuberculosis, that's the main one of the mycobacteria that is a threat. It used to be called consumption in the old days, because people almost looked consumed by the disease. They would just get thinner and thinner. Literally it was a wasting disease. People would be sent up into the mountains of Switzerland to try to recover from consumption, to where the air is clearer and cleaner, and maybe hope that they can recuperate.
But TB-- look at these numbers. In 2015 there were almost 10 million new cases. There are about 1.2 million deaths from TB. A serious situation with TB is that it's often found co-infecting with the HIV virus, where you just can't fight the TB. So eventually, if you're infected with the HIV virus, it's the TB that gets you due to the weakening caused by the infection with TB.
So these numbers are shocking in light of the numbers I showed you on the previous slide, right. Look at these numbers if you go to snakes and things like that. They're meaningless numbers compared to infectious diseases.
So now, and I'm going to talk to you about the origins of this, many, many infectious agents that we thought we had conquered-- we thought we could take care of it. You just take this course of antibiotics and you're off, you're set. But now, because of the rapid mutation rates in bacteria and viruses, certain pathogens have completely worked out mechanisms to escape therapeutic agent. And I'm going to talk to you about those mechanisms towards the end of this class.
So basically you can dose a person one day with a normal dose of an antibiotic agent, and then 10 months later that normal dose or 10 times or 100 times that dose stops working. Why is that? It's due to resistance acquisition due to rapid cell division and mistakes made on replication and transcription, that then may one in a million times confer an advantage on the microorganism. All of a sudden the drugs don't work anymore.
The WHO and various community notice boards call this set of infectious agents the escape pathogens. It helps us remember which ones these are, because these are pathogens that escape treatment, because they've developed resistance to multiple drug cocktails. So commonly, when someone has a particular disease they don't take one drug, they take two or three to hit lots of pathways at once in the hope that resistance won't develop fast. But the escape pathogens have collectively acquired resistance to several antibiotics, meaning there's no good treatment. So the letters of escape stand for Enterococcus faecium, Staph aureus, Klebsiella pneumoniae, Acinetobacter baumannii, Pseudomonas aeruginosa, and some of the Enterobacter species.
Some of these infectious agents are what result from-- I always say this wrong-- nosocomial infections. Does anyone know what those are? These are infections that people get in hospitals. So Tom Brady had a knee operation. He got an infection in his knee that came from the surgery, right. These are hospital acquired infections, because you can't sometimes clear an area enough, and there's infectious agents around.
So Acinetobacter baumannii was dubbed the Iraqi Bug for many, many years, because the vets coming back from Iraq were going to military hospitals, and these were abundant with cases of Acinetobacter baumannii. So that moved on to the escape pathogen list. So these are things to watch out for.
It's the reason that nosocomial infections-- I hope I'm saying it right, otherwise you're going to go off and Google it and realize I said it wrong. It's the reason why old school physicians wore bow ties and not ties. Can you imagine why? So if you're wearing a tie, which I seldom wear to be honest, and you're working over a patient, the tie can be the thing that carries the infection, because it gets closer to infected areas. This is old school stuff. And so originally the physicians wore bow ties in order to distinguish themselves as important people, but not to wear ties that might carry infectious agents. That's sort of a scary thing.
So with all this said, let me just lead you in to talk about bacteria antibiotics and resistance development. So we often name bacteria somewhat by their shape. So the long rod shaped ones are cocci. The round ones are cocci. The rod ones, whatever they are, come on one of you. And then what did the rod shape ones? Bacilli. I had a blank moment. So the rod shaped ones are bacilli. And then there's some others that have a different morphology like Campylobacter jejuni that have kind of a corkscrew shape. And that's thought to be important in their motility, digging through the mucous layers in the epithelial layers. So here I show you several shapes of bacteria.
And I'm just going to, once again, reinforce what diseases some are associated with and some other diseases that you might be surprised by. So yes, we know about salmonella and the E. coli and food poisoning. But Helicobacter pylori, which is one of these flagellated bacteria, can infect the stomach. It's often the cause of ulcers. So it's a causative agent of stomach ulcers, but that has in turn led to a considerable risk factor in stomach cancer. So what we thought was just an infection causes a constellation of other problems, including cancers. And more and more microbial agents are now associated with cancers, in particular the viruses.
Neisseria, these come along with the sexually transmitted diseases such as gonorrhea. Neisseria meningitidis is the one that causes meningitis. It is a very, very often fatal infection of the meninges. Staph aureus lots of infections around the body, just gruesome things like cellulitis, wound infections, toxic shock. Streptococcal bacteria, I've already mentioned-- the pneumonias, and then Campylobacter.
And now another complicated factor of infection-- so I talked to you about stomach ulcers and stomach cancer. Another thing that seems to be coming along with infections is autoimmunity. So in the last section of the class you heard about immunity and you also heard about tolerance, that we don't react to things that are ourselves, otherwise we'd be in deep trouble. Autoimmunity can suddenly pop up from certain bacterial infections, because bacteria tend to cloak themselves with unusual sugar polymers and other kinds of structures that the body doesn't really know what to do with.
And in some cases they kind of mimic things that are in the human body. So they they are mimetics of normal structures in the human body. And the body just doesn't notice them at all. And then there are incidences where certain bacterial infections later on cause autoimmune disease. So a bacteria may come along. It may have something that looks kind of like something human, but not quite. The human body responds, develops antibodies, and then they cross talk back to aspects of our physiology.
So Campylobacter jejuni is often a contaminant in poultry. It's a severe GI infection. But later on people get diseases such as Guillain-Barre, which is a neuropathy where the ends of your limbs become numb and non-functional. So there was a famous football player, the one they called "The Refrigerator," who had a serious case of Guillain-Barre resulting from very much an infectious disease, which converted into autoimmunity.
So let's now look at antibiotic targets. And to look at antibiotic targets, I think the first clear place to look is at the bacterial cell wall. Now when we first started talking about prokaryotes, things that include bacteria, we talked about the fact that these single celled organisms have to have a robust cell wall to prevent osmotic shock. They have to have some kind of thing to keep them from taking up too much water and basically exploding because of osmosis. Water floods in to balance the salt concentrations. So they have a complex cell wall, which is made of a macro molecule called peptidoglycan And it's usually one word, but I want to just underline peptidoglycan because it's a fascinating polymer that's made up of peptides and linear carbohydrate polymers.
So if you look at this typical bacterium, this is just a cartoon of the peptidoglycan. So it's a cross-linked polymer, we in one direction it has repeating carbohydrate units. I'm not drawing those complex hexose structures there. I'm just drawing it in cartoon form.
And those are carbohydrates known as NAG and NAN. NAG is N-Acetyl Glucosamine. It's a hexose sugar. NAN is N-AcetylMuramic acid. It's another modified sugar.
And on the one of those sugars, there is a reactive site that allows you to basically cross-link these polymers into a mesh work. So it's a feat of engineering to build this amazing polymer. It starts being built on the inside, on the cytoplasm. And then the components get flipped onto the other side of the cytoplasm of bacteria. Then they get polymerized in place to make this complex mesh work of a polymer that creates the rigidity of the bacterial cell wall.
It's generically known as a peptidoglycan. Different bacteria have different peptidoglycans. There are several modifications that might be specific to particular bacterial sera But this is the generic structure, where you have a polymer that's built of sugars. You can recognize the sugar structure there going in one direction and the peptide component that cross-links across in order to make this mesh.
And bacterial wall have different amounts of this, but it'll build up to a really strong, rigid mesh work that's permeable to things, small molecules and water. There is holes, and so on. But it creates a mechanical rigidity so that osmotic shock doesn't occur on the bacteria. Any questions about that? Does that makes sense? So that, in a sense, it's their exoskeleton, if you want to think about it like that.
So the properties are rigid. Without it, the bacteria would suffer osmotic shock. And it's plenty permeable to allow 2-nanometer type pores in order for nutrients and water to go into the structure.
[VIDEO PLAYBACK]
- --have E. coli growing here. And it's living. You can see it start to grow. Here we add penicillin. We're going to see these bacteria--
PROFESSOR: These are bacteria, rod-shaped bacteria.
- There wasn't any microphone on this, so--
PROFESSOR: And I'm going to ask you to just keep watching this kind of carefully.
- There goes another one, boop, boop.
[LAUGHTER]
- Poking holes in the cell wall, boom, bacteria is dead.
PROFESSOR: Look at some of the bacteria disappearing. All right, I guess
[INTERPOSING VOICES]
OK, then we're going to--
[INTERPOSING VOICES]
So we're going to leave it.
[END PLAYBACK]
Let's go back one. OK, now what was that? OK, so I've told you bacteria would suffer osmotic shock without peptidoglycan. Those are bacteria that you see popping, as the person who was talking said, because the peptidoglycan cannot be made. There is an antibiotic that's added. It is penicillin that's added to the bacteria.
And it stops-- as bacteria grow, they have to make a bunch more peptidoglycan, because if you're doubling, you've got to make twice as much peptido-- you've got to double the amount of peptidoglycan. If you have something that inhibits the peptidoglycan being made, you have a bacterium that's trying to stretch out what it has, it's not resistant to osmotic shock. And what you saw was the bacteria basically undergoing cell death via osmotic shock, pretty graphic, pretty visual.
So penicillin was one of the first antibiotics that was described for the treatment of bacterial infections. And we'll go to the timeline of that in a moment. So when we talk about bacteria, the original definition of bacteria is in three different subtypes, gram-negative, gram-positive, and mycobacterial.
This is actually the first way that people would take a look at your cell-- at the bacterial cells and diagnose roughly what kind of bacteria they were. Did they fall-- which of these broad families did they fall into? Because it would help in defining how you would treat the infectious disease.
So I want to show you the difference between the cell wall of these various types of bacteria. And the truth is, if you have an infectious disease, your wish is, if you had to pick one of the three, that you have a gram-positive disease. And I'll explain why that is in a moment, because it's all to do with how drugs can get into the bacteria to inhibit vital functions in order that they die and they don't take over your system.
So let's look first at gram-positive bacteria. They're shown here. This is a section of a bacterium. Gram-positive have a single cell wall. And they also have a thick layer of peptidoglycan.
So they gain rigidity by basically having an extracellular thick layer of peptidoglycan coating them. There is a schematic of it here. So here would be the inner cell wall. And here would be the peptidoglycan, shown in orange and pale, buff-colored circles. So that would be where their peptidoglycan is.
And then there are some other glyco conjugates that actually stick out beyond that. But there is only one cytoplasmic membrane. That's the standard double bilayer.
And the peptidoglycan is quite thick, relatively, 20 to 80 nanometers across. So that's how wide it is. And you can, if you've got a-- if you've stain a bacterium under a microscope, you would see that, the thickness of that wall, but the absence of a double wall.
The gram-negative bacteria have a double wall. The inner membrane is pretty standard. It's just typical phospholipids. It looks like the inner cytoplasmic membrane of the gram-positive bacteria. And then it has an outer wall.
So the inner membrane is typical. And then the outer wall has one leaflet that looks kind of normal. And then it has a second leaflet that's sort of decorated, honestly, like a Christmas tree.
There is all kinds of things sticking out there that interact with hosts that they infect, and so on. And the space between the two walls is called the periplasmic space, because it's between. It's not the cytoplasm. It's what's called the periplasm.
Now, what's interesting about these, the gram-negative bacteria, is they have quite a bit less peptidoglycan, only about 7 to 8 nanometers. So that's pretty interesting. But they sort of gain robustness from that second wall structure that's coating on the outside.
Now, their challenge with gram-negative bacteria relative to gram-positive bacteria is any drugs you develop have to make a pretty-- if they're targeted at intracellular sites, they have to get through two walls, not just one wall. So they are harder to treat. And they also have a lot of characteristics that make them more prone to resistance development.
So I want to point out to you, on this electron micrograph, you can actually see the double wall, the dark band of space and then another dark band, whereas here you see a thin single wall, but you see a lot of junk on the outside. Is everyone seeing the differences just to look at them?
OK, so what's this gram thing about? What does this stand for? It simply stands for a chemical dye that stains peptidoglycan. And it was invented or discovered by Professor Gram. That was his name. So when someone says you got a gram-positive infection, gram-negative infection, it's how those cells look when they've been treated with this stain.
Gram positives show up very positive to the stain because there is a lot of peptidoglycan on the outside that absorbs the dye and shows a strong color. The gram-negatives don't show very well with a Gram stain, because the peptidoglycan is tucked in the periplasm, not on the outside of the cell. So if someone does a quick check on a bacterial streak or an infection that you have, they might treat it with the Gram stain and say gram-positive or gram-negative just based on that simple color analysis. And so in one case, the peptidoglycan is abundant and accessible. In the other case, it's very, very much thinner and less accessible to the dyes.
Now, this probably looks like stone-age stuff to you, because how much can you learn by these simple colorimetric stains? We're certainly moving in very, very different directions. But let me just finish off with the third type of bacteria, the mycobacteria, which include Mycobacterium tuberculosis.
And they have a different kind of wall, again. And they're pretty unusual. And they are really, really hard to treat, because it's almost impossible to get therapeutic agents into mycobacteria.
I used to work on a team with Novartis in Singapore. And they said, doing anything with mycobacteria was like trying to do biochemistry on a wax candle, literally. You just can't work with it, because they have a thick additional wall that's kind of different again. Did you have a question? No. Sorry, I thought I saw your hand up.
So what they have is a typical cell wall then some peptidoglycan, but then they have this thick mycobacterial layer which comprises what are known as mycolic acids, which basically add this thick layer of greasy hydrophobic material on the outside of the mycobacteria that's pretty impenetrable. The cell wall is quite different. It doesn't have an outer coat. It's like gram-positives in that respect.
But it doesn't stain very strongly. So it has a weak, what's known as Gram stain. So sometimes if you've got something that gives a sort a so-so response to the Gram stain, you might say, oh, it looks like a mycobacterium because of what's happening.
Now, mycobacteria TB is a huge threat, because its treatment, its current treatment-- and it's the same treatment that's been around for, like, 30 years or something-- is a treatment with four different antibacterial agents that hit a bunch of different sites in the lifecycle of the bacteria. It includes these compounds shown here which are isoniazid, rifampicin, ethambutol, and pyrazinamide. And it's a six-month treatment with those medications, so handful, four different medications for six months.
So what they were realizing in the developing world is that there was terrible compliance. The drugs are cheap, but there was no compliance. People just were not taking the pills, because they're like, I'm tired of taking these pills every day for six months.
So what was developed was what's known as the DOTs program. Has anyone never heard of this? Is anyone interested in infectious disease? It was a situation where it was a social system set up in order to make sure people took these drugs every day for six months in order to comply.
So social workers would go to the villages in remote areas and watch people take the medications. So it's directly observed treatment to make sure they followed through, because if they had regular TB, not very resistant TB, you could overcome it, provided that you took these medications. But still, it's a hugely debilitating thing to have to deal with these treatments.
Now, there are two strains of TB. One is called MDR-TB. And the other ones called XMDR-TB You'll occasionally hear of these on TV programs. MDR is resistant to three of the four medications. And XMDR, which stands for extremely MultiDrug Resistant, is resistant to every single one of those medications. New medications, different mechanisms of action are sorely needed.
All right, this is just what things look like with the Gram stains. So here you see gram-positive Bacillus anthracis. That's the deep purple rods. You know that's a gram-positive because it's a deep purple stain.
The other cells in this picture are white cells. So you can really pick out the gram-positive. This is the structure of the chemical dye that stains peptidoglycan through absorbing into the peptidoglycan. It's a very sort of physical interaction of the dye with the polymer.
And over on this slide, it's a mixture of gram-positive and gram-negative. And you can pick up the gram-positive and differentiate them from the gram-negative, which just stains sort of kind of weakly pink. And then mycobacteria, which are formerly gram-positive, don't stain very well because of that thick mycolic acid hydrophobic wall.
So what would you do nowadays? Would you pull out a stain and drop it on bacteria and get some vague response? What's open to you now in the 21st century? You have a tiny sample of a bacterium. Grow it up. What would you do? You could tell exactly what it is.
AUDIENCE: PCR.
PROFESSOR: Yeah, you'd PCR up the genomic DNA and then go match it, because the thing that we, in addition to the human genome, there are thousands of pathogenic bacteria sequences that are completely annotated, known. The [INAUDIBLE] has a massive compilation of these sequences. And you just go and you find out what the bacterium is based on the sequence.
So now rapid sequencing efforts-- maybe they're just a few number of key places in a genome that you would go towards and just do a really fast array and figure out what's there and within what bacterium it is, which gives you a much better clue as to how to treat it than the vague, ambiguous stains. So even though stains keep going, there is now other ways.
Unfortunately, not everyone has the instrumentation to do rapid sequencing. So nowadays, there is a lot, lot, lot of interest in faster dipstick sorts of tests that can distinguish between different bacterial strains by, for example, interrogating that coat of glyco-conjugates that's on the outside of the bacteria, dipstick paper tests that can give you an idea of what organism and what serotype so you can move forward and do a much more rational treatment of those organisms.
OK, let's see what's-- yes. All right, so where did the antibiotics first come from? Any questions so far? OK, so where did the first antibiotics come from? From a couple of accidental discoveries. Who has heard of the Fleming experiment? Who knows about that discovery of penicillin?
Yeah, so there was an original observation that predated that which sort of suggests that Pasteur was a pretty smart guy, because he contributed in a lot of different areas. He discovered that some bacteria tend to release substances that kill other bacteria. That was in the 1870s.
Then later on, there was another sort of spread of antibiotic agents. And it came with the discovery that we had things like arsenic derivatives actually showed some value in treating the organism that causes syphilis. So talk about the treatment being-- the cure being worse than the infection. People were being treated, seriously, with these arsenic derivatives in the hope of wiping out the infectious agent that caused syphilis. But you know, sometimes it was a mixed bag.
But where things started to get a lot more interesting was that in 1928, there was this sort of famous historic story of Fleming discovering that some bacteria seemed to be inhibited by a particular agent that came from a fungus. And this was the origin of penicillin. So he would have a Petri dish where he was growing bacteria. And he noticed that in some of his samples, there was inhibition of bacterial growth due to an exogenous agent that had somehow contaminated the plates.
So in that story, that was the substance that was named as penicillin. The mold from the-- mold is the fungus-- actually inhibited the growth of staphylococcus bacteria. And it was called penicillin. And then a lot more time went by.
But in the 1940s, the active ingredient was discovered. So 1940s is sort of slap bang about, I would say, a couple of years into the Second World War. And they were able to mobilize the production of this agent.
Towards the later end of the war, people had penicillin available to them. And it's basically pretty well believed that, if it wasn't for the antibiotic agents that emerged-- you know, the war ended in 1945. If it wasn't for those agents that emerged, there would have been way way, way more deaths from the war. As it was, there were way too many.
So penicillin was the first antibiotic that was discovered with a discreet mechanism of action. And it was discovered at a very, very important time. So that was all great news. Penicillin was produced widely. Some of you may be allergic to penicillin. There are other options nowadays. But it's the cheapest and most viable of the first-line antibiotics.
Here we go. And this thing, this pointer has a mind of its own. It sort of changed its mind.
But the problem was the bacterial species started to survive treatment due to development of resistance. And all of a sudden, something that worked really well wasn't working anymore. So let's try and think about peptidoglycan, what penicillin looks like and what it does, and how penicillin resistance emerges. Those are the three things I'm going to cover here.
OK, so what does penicillin do? Penicillin stops the formation of this big macromolecular peptidoglycan polymer by stopping the last cross-link, stopping the chemistry that happens to join the peptide chains to make a cross-linked polymer. And anyone who is in the mechanical engineering area will know that polymers that are just strands are much weaker than polymers that are crossed-linked structures which have tensile strength in both directions.
So the uncross-linked peptidoglycan was weak. And what penicillin specifically did was inhibit forming that cross-link. What does penicillin look like? Here it is. It's a cool structure. It's what's known as a natural product, five ring, four ring, an interesting structure. And what it would do is it would interact with the enzyme the cross-linked the peptidoglycan and basically stop it dead in its tracks.
What did the bacteria do? The key part of this structure is this four-membered ring within amide bond in it. The bacteria evolved an enzyme to chop it open basically making it completely inactive. So beta lactamase was evolved in the bacterial populations.
It was probably derived from some other enzyme that did some useful function, but not targeted to the penicillins. But the bacteria started to survive because they made a ton of an enzyme called beta lactamase. And then it completely stopped working.
So the chemists came up with other options, because they said, well, you know, if that doesn't work, we've got other antibiotics in our arsenal. And there is a compound that was used for years as a last line of resort antibiotic known as vancomycin. It was very, very important, so very serious infections, and really preserved for that use. And they thought that vancomycin might be a drug that just couldn't be defeated.
This big molecule here is vancomycin. This little piece of peptide is actually the peptide that's in that cross-link. And vancomycin basically, like a glove, sat on that piece of peptide and stopped it being cross-linked. And what did the bacteria do? They evolved a set of enzymes to completely change that little piece of peptide into something that bound more poorly, giving you resistance to vancomycin as well.
So when there is one drug involved, it's pretty easy to get resistance quite quickly. You just mutate one enzyme and you get a resistant strain. And the enzyme that can beat the antibiotic will win.
If you've got a compound that takes five different enzymes or an antibiotic that has a very complex mechanism of action, you might say, well, this is never going to be defeated. It took five additional enzymes to evolve to make the peptidoglycan a different structure. And it's not that within every bacterium, you mutate five different enzymes and get them all working as a team. What was happening in these infections is that a plasmid with the set of enzymes was being passed around amongst bacteria. So a new bacterium could acquire resistance to this compound without evolving a whole bunch of new enzymes, but rather by lateral transfer of plasmids encoding the genes that it took to make the vancomycin inactive.
All right, so let me just tell you a few of the targets. And then there is one movie I want to show you that's kind of cool. So currently, when we inhibit bacteria with antibiotics, there are a number of essential processes that are targeted with common antibiotics.
So this would be a typical bacterium. One target of action is DNA synthesis and DNA polymerase. And the enzyme that is targeted is one we've talked about, topoisomerase. And that is inhibited by the fluoroquinolones such as ciprofloxacin that actually targets specifically the bacterial polymerase. So that's one way, inhibit DNA replication, bacteria can't divide.
Another set of antibiotics are those that inhibit protein synthesis. So in particular, you know the tunnel that comes out of the ribosome where the growing polypeptide chain emerges after reading the messenger RNA and translating the messenger into protein? There are antibiotics to basically stick in that tunnel and stop protein synthesis. And those are things like the aminoglycosides. And they block exit from the ribosome. But you could imagine that mutating.
There are the ones that inhibit cell wall biosynthesis that I've already talked to about, the penicillins, the vancoymcin. And then there are others that inhibit folate synthesis. And then there is a lot of synthetic drugs, but also a lot of natural product drugs. So both nature and chemistry have teamed up to inhibit all of these essential steps.
OK, so how do you test for antibiotic resistance? You use plates where you're growing particular strains of bacteria on a plate. This would be a colony. And it's growing outwards.
Where there is a colony but there is no growth around it, it means there is something in that plate that is inhibiting bacterial growth. So these are very clear types of ways that people check to see if bacteria have become resistant to drugs. You would look for that zone of inhibition. Does it disappear with some of the resistant strains, for example? And these get pretty sophisticated now where you can test a bunch of antibiotics in one go, where each of these colored dots represents an area where there is treatment with one antibiotic or another.
So what's the problem? The problem is this graph, that as soon as an antibiotic is introduced, just a few years go by. And there is resistance to that antibiotic.
So resistance basically is the gradual acquisition of machinery to somehow inactivate the antibiotic treatment. So if you take a look, here on the top is where the drug is introduced. And on the bottom is when resistance was developed.
So let's go to something we're familiar. Here is penicillin, people introduced about 1940 to the general population. By about '47, there was resistance to penicillin. And you can see, this is really just a really serious sort of series of events.
So what I want to show you was resistance in action. And that'll be the last thing I talk about today, because I just want to give you a feel for what does resistance look like. So this was an experiment that was done at Harvard on just a visualization of resistance development.
I think what's so fascinating is you could then go back to the plate and pluck the first pioneers who crossed that line and find out what that was. What was that mutation that let the population expand, and so on? So you could really map out the entire evolution of very, very strong resistance.
So in the next class, I'll talk to you about resistance mechanisms. And then we'll talk about viruses and resistance to antivirals.