Flash and JavaScript are required for this feature.
Download the video from Internet Archive.
Description
Professor Martin explains the body's own barriers to cells becoming cancerous. He then discusses how cancer spreads to the rest of the body through the breakdown of growth/survival signaling, cell-cell adhesion, and cell motility.
Instructor: Adam Martin
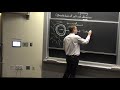
Lecture 26: Cancer 2
ADAM MARTIN: So I guess I will start by, first of all, congratulating you all. Since the last time I've seen you, you've all regenerated your intestine. So it's like you're a brand new person. So there's that. I want to start the lecture by talking about something I've been putting off telling you about, which is the Nobel Prize in physiology or medicine that was awarded this year.
And it was awarded to James Allison and Tasuku Honjo for their discovery of a way to harness the immune system to fight cancer. And we're going to talk about the immune system later in the course, and it turns out, I will lecture on it. And I've actually had immunology, and in fact, I had immunology with James Allison.
So I will do my best to channel James Allison when I talk about the immune system. But what they won the prize for is, they figured out a way to essentially release the brake on the immune system in order to allow the immune system to better fight cancer. And this is a technique that's been used in the clinic, and there are currently a number of clinical trials that are also looking to see whether this can be used in a variety of different cancer types.
OK. So I just wanted to point that out now because we're talking about cancer, and I'll go more into the mechanism as how this works when we start talking about the immune system. So as I mentioned in the last lecture, cancer is basically a progressive loss of tissue organization.
And luckily for us, our body has a number of different barriers to cells becoming cancerous and forming a tumor. And so I wanted to start out just by having you guys tell me what you feel the barriers would be to this process. So who has an idea of a barrier? What are the barriers in place by your body to prevent cancer cells from arising and forming a tumor, and for this process to form a malignant tumor? What's that? Oh, Rachel, sorry.
STUDENT: Apoptosis.
ADAM MARTIN: Apoptosis is a good one, right. So there's a careful process in your body to limit how long certain cells are resident in the body. And all of this depends on communication between different cell types in your body-- so barriers to tumor agenesis. And one type of signal is a survival signal. And if a cell is not getting a survival signal, then the cell will undergo apoptosis, which is what Rachel was referring to.
So one barrier is that there is a highly regulated system of determining whether or not cells divide and also whether or not cells live. OK. So there are growth survival signals, which means that the decision for a cell to go off its rocker and just start dividing uncontrollably is not likely for a normal cell. Something has to happen to that cell in order to perturb it.
So what are some other barriers to forming a tumor and for that tumor to become invasive? Yes, Jeremy?
STUDENT: You just mentioned it, but the immune system.
ADAM MARTIN: Yeah. So this cancer cell can't activate the immune system. So you could have some type of immunosuppression of the cancer. And I won't talk about that today, but maybe we'll come back and talk about it when we talk about the immune system. OK.
Looking at this diagram, when the tumor proceeds to invasive cancer, what do you think are some barriers to that process happening? What are cells normally doing in an organ? The cells that line your intestine, are they invading into the surrounding tissue? No. Jeremy is shaking his head no, right?
So normally, cells don't do that, or certain types of cells do, but normally, if you have cells that are the lining of an epithelium, they're not going off to a blood vessel. OK. So I'm just going to review the structure of the tissue, and then we'll talk about some of the barriers that prevent this from happening.
So I'm drawing a tubular organ here. This could be the tube of the intestine. There'd be a lumen inside here. It could be some ductal structure in an organ, like a mammary gland. It could be that this is the airway of a lung, and there's an epithelial lining around that airway.
So these cells here are forming an epithelial lining. And one important aspect of epithelial biology is, immature epithelia, they have a floor that the cells stand on. So this structure that I just drew around these cells in green is known as the basement membrane.
It's the basement because it's the floor that this other thing is built on. And this basement membrane is made out of what is known as the extracellular matrix, which I'll just call matrix right now, and the extracellular matrix are basically extracellular proteins that form a mesh work that forms a rigid structure on which epithelial cells can sit on.
OK, now if we think about what surrounds this organ, there's also matrix proteins in the intervening areas. And this is known as connective tissue. And so if we think about the cells that are the epithelial cells here, they are in one state, and this state is known as epithelial, as I just said. So these are epithelial cells.
Epithelial cells have a few properties that are really distinguishing. The first is that they have high intercellular adhesion. And the next is that, if you consider their relative mobility, they can obviously move within the epithelium because we talked about the intestine and how cells are moving from the base of the crypts up to the villus.
But in general, these cells aren't moving in and out of the organ. So I would say that they have a low migratory potential. And so these are the epithelial cells that are here. Now there are also cells that are in this interstitial space, and I'll draw-- they often have this highly elongated morphology. And these cells are in a fundamentally different state.
And this state is known as mesenchymal or stromal. So this area outside the organ is also known as the stroma. So they're known as stromal cells in this location. And these cells have very different properties than the epithelial cells in that they have low adhesion-- I should say low cell-to-cell adhesion.
And in contrast to these epithelial cells, these stromal cells, as you see in this example up here-- that's a human neutrophil-- these stromal cells are highly migratory. So that neutrophil is chasing a bacterium, and they'll eventually get it, but the movie will loop, and so you'll see it constantly chasing that bacterium.
So some examples of stromal cells would be immune cells, like this human neutrophil. And you probably know that immune cells have to traverse different organ systems. They have to be rapidly recruited to sites of infection or sites of injury. And so this type of cell is fundamentally different from the types of cells that line your organs, where you basically need those cells to stay put.
When considering cancer, you have these different types of cells in your body. 90% of cancer comes from a cell of epithelial origin. So 90% of cancers are epithelial in origin. Some other examples of stromal cells are cells like fibroblasts. And fibroblasts are cells that secrete and remodel the matrix that's in connective tissue. And they're also important for wound healing and secreting matrix during the wound-healing process.
So that's just to give you a few examples. OK. So now let's come back to this example and talk about what happens to get a cell to go all the way from being a normal epithelial cell to having this type of behavior, where initially you have growth and a loss of the control over growth and survival signaling, and eventually, the cancer can become what's known as malignant?
So it's a progression, and initially, you might just get increased in-cell division and abnormal cell shape, which is known as dysplasia, and at that point, it's known as something like an adenoma or something that's benign. But it's this last point here, up here, where the cells breach this basement membrane. When that happens, then the cancer is known as carcinoma, and it's malignant. So malignant cancer is cancer that has breached the basement membrane.
So I want to take you through the progression of tumor genesis. And first, I'm going to start with the breakdown of this growth survival signaling. And I just wanted to remind you about what we talked about with the intestine as our model organ. And in the intestine, remember that this is all regulated by signals between stem cells and niche cells.
So the niche cells are sending the stem cells signals like Wnts that control their self renewal, and then it's the loss of this signaling that allows these cells to eventually undergo apoptosis and get shed into the lumen of the intestine.
So one of the first steps in cancer is this breakdown in growth survival signaling. So this first breakdown is going to enable the cells to overcome this first barrier. And so as I just pointed out, remember, it helps to think of what has to happen in a normal tissue or organ.
Normally, the decision whether or not a cell divides or dies-- so cell division and also death-- is highly regulated. And it's regulated by communication with other cells. So in cancer, this regulation goes awry. And how would this regulation go awry? Yeah, Jeremy?
STUDENT: Loss of function in a tumor suppressor or an over-activation of the oncogene.
ADAM MARTIN: Mm-hmm. So Jeremy suggested that there could be mutations, like oncogenic mutations or tumor suppressor loss, that lead to abnormal cell division and death. And that is exactly right. So you could have oncogenic mutations, and these oncogenic mutations, they often hyperactivate or constitutively activate these growth-signaling pathways that are normally downstream of growth factors and receptor tyrosine kinases.
And if you hyperactivate those, you reduce the dependency of the cells on these signals. So these oncogenic mutations can reduce the dependency of cells on growth factors and growth signals. OK. But one important point is that this is not enough. And you can think about this in the case of the intestine because if you had an oncogenic mutation in one of these cells here, it might not be as dependent on signals for growth.
But it doesn't matter because even though you had that mutation, it's going to die, and it's going to shed out of the tissue. And actually what happens in many cases where you activate a signaling pathway that allows the cell to, in an abnormal way, go through the cell cycle, you actually induce a failsafe mechanism at the cell which causes the cell to undergo apoptosis.
So often, you get this step happening, and the cells just undergo apoptosis because you've evolved to protect your organs from this type of mutation. So it's the oncogenic mutation, in collaboration with loss of tumor suppression, and one of the main tumor suppressive mechanisms our body has is apoptosis, where if a cell is doing something abnormal, the cells simply dies.
So loss of the tumor suppressor could be loss of a gene that promotes apoptosis, and in that case, if the cell loses that mechanism, the cell will avoid apoptosis. OK. So this is oncogenic mutations and the loss of tumor suppressors which subverts the normal communication between cells which is required for normal tissue homeostasis. OK.
Now another example of this growth and survival signaling is not one which involves, necessarily, a genetic change, but one that involves changes in expression. And it also involves interaction between the tumor and the surrounding cells of the body. So I'm going to tell you a little bit about tumor microenvironment.
And this is something that's important to consider in cancer because a tumor in a body, it's not in isolation. It's surrounded by other cells in your body and other things in your body, like matrix, and so here is a picture showing you a tumor, and the tumor is stained with this membrane protein which is shown here in the rust color. So that's the tumor.
And what's also stained in this piece of tissue is the DNA. It's stained in blue. And so you see the nuclei of the tumor cells in there. But you see all these blue nuclei surrounding the tumor. And these are stromal cells that are around the tumor. And they've actually been recruited to the tumor by the cancer cells.
So what I mean by tumor microenvironment is just the region around the tumor. And so if you have cancer, and you have a tumor, the tumor cells can actually secrete signals which recruit stromal cells. So there can be an interaction where the tumor sends recruitment signals that causes stromal cells to come by.
And what the stromal cells can do, what they do for the tumor and why this is beneficial for the tumor, is that stromal cells can secrete growth signals or survival signals that promote the growth of the tumor. So there can be a reciprocal interaction here where you get an abnormal conversation between cancer cells and the surrounding stromal cells.
But again, this is very similar, if you think about it, to the way a normal organ works. You often have these signals going between cells. And that is involved in normal tissue homeostasis. What's happening here, though, is abnormal and that the cancer cells are constitutively recruiting these stromal cells just to get this growth signal that they're addicted to.
And because you have just the presence of such a loop suggests that these cancer cells, even if they have oncogenic mutations and have lost tumor suppressors, they are not totally independent of growth signals. They still, to some extent, rely on growth signals. So these oncogenic mutations, they reduce the dependency on growth signals, but this dependency, the dependency on growth signals, is not eliminated.
And one experiment that showed this was an experiment that was done back in the 1950s where patients with a type of skin cancer, basal cell carcinoma, were taken, and they had their tumors excised from one part of their body. And then the tumor was grafted back onto them, to another part of their body, distant from the original site of the tumor.
And this grafting experiment was done either with the stromal cells that surrounded the tumor or the stromal cells surrounding the tumor were removed, and just the tumor cells were grafted back onto the body. OK. Now this experiment probably would not be allowed today, but back in the '50s, I guess it was legal.
And so the experimental result is that if you graft the tumor cells with the surrounding stroma, the tumor was able to establish a second tumor, or the tumor cells survived this grafting procedure, whereas tumor cells that were taken without the stroma underwent cell death when they were put in a new location.
So there's something about this specialized microenvironment that the tumor creates for itself that is enabling the tumor to grow and survive. And the model is that it's because these stromal cells are secreting growth factors that these cancer cells are still dependent on. So this dependency on growth signals is important, clinically, because some types of cancer, there is an elevation of growth factor receptors that are associated with the cancer.
And the famous example of that is, in 30% of breast cancers, there is a growth factor receptor, the HER2 receptor, which is over expressed in the cancer cells. So I just drew a receptor here, and I'm now talking about the HER2 receptor. HER2 stands for human epidermal growth factor, EGF, receptor 2.
So this is a receptor tyrosine kinase, which is a transmembrane protein receptor. It's expressed on the surface of the cell. And so about 30% of human breast cancers are HER2 positive, which means that the cancer cells are over expressing this receptor tyrosine kinase.
The fact that you have these cancer cells over expressing a growth factor receptor suggests that the cancer requires some type of growth stimulus in order for the cancer to be growing. And the fact that this was discovered, that 30% of breast cancers over express HER2, has been used by researchers to develop a treatment for this type of cancer, this HER2 positive.
The treatment is known as Herceptin. Possibly some of you have heard of this. It was developed at Genentech. And what Herceptin is, it's an antibody that was raised against a human HER2 protein. OK. So Herceptin is an antibody. It recognizes HER2 on the surface of these cancer cells, and you can treat patients with this antibody, and an antibody binds to the HER2 positive cells.
And it either blocks the function of HER2 or recruits immune cells to kill those cells off. The exact mechanism, I don't believe, is known. But what is known is that Herceptin has really changed how we're able to treat HER2 positive breast cancers. And it's been a huge success story in the fight against cancer.
All right. So we've talked about this first barrier, the barrier to tumor cells becoming at least semi-independent of these growth factors. And so now I want to talk about other barriers to tumor genesis. And if you consider an epithelial cell here, even if these cells are able to grow, they won't be able to leave the organ until something else happens.
And one thing that would need to happen for these cells to become malignant and to leave the organ that they were initially part of is for there to be a breakdown in the adhesion between cells. So for the next few minutes, I want to talk about the breakdown in cell-to-cell adhesion.
So how would a cancer cell basically unstick itself from the cells that surround it in order to leave an organ and go somewhere else? And for that, to explain that, I have to remind you about some of the normal biology of these epithelial cells, which we talked about earlier, which is that they express these transmembrane proteins that are adhesion proteins.
So normally, epithelial cells have adhesion proteins. And the famous one for epithelia, or one of the famous ones, is called epithelial or E-cadherin. And E-cadherin is a transmembrane protein. It has an extracellular domain, but rather than that extracellular domain binding to some secreted ligand, this extracellular domain recognizes E-cadherin molecules on other cells, and they stick to each other.
And it essentially functions like cellular Velcro. So the cells link together, and they stick to each other such that they form a coherent tissue. So the way that cancer cells subvert this mechanism of adhesion is, they have to do something that inhibits E-cadherin. And so cancer cells, what they can do is to decrease the cell-to-cell adhesion, and I'll tell you how in just a minute.
And in addition to decreasing this cell-to-cell adhesion, they can also promote the genes that are involved in motility. So we have to understand, then, how it is a cancer cell would do both of these things. And I'll start with cell-to-cell adhesion.
And in contrast to what we've talked about with the growth and survival signaling, where you have mutations that happen in cancer cells, and you basically have an irreversible change to the genome of the cell, this change in the adhesive properties of the cell, and essentially their cell state-- because what you see here, if a cancer cell is having less adhesion and getting more migratory, it's switching from an epithelial type of state to a mesenchymal type of state.
And what this is called is an epithelial-to-mesenchymal transition, and that is EMT, for short. And this EMT is not a genetic change. It's not caused by a genetic change, but it appears that EMT results from changes in gene regulation.
So you can think of it as more of an epigenetic change, an epigenetic change in gene expression. So there's a change in gene expression such that this can reverse later on. And there are master regulators of this process, which alter gene expression. And so the type of gene that alters gene expression, these are often called transcription factors.
And there are several transcription factors that are master regulators of this EMT process. And their names are Twist, Snail-- another one's called Slug. There's an Escargot. You can see this got a little out of hand. And you can tell by the names that they probably were not discovered in humans.
And in fact, these genes were discovered in the same genetic screen that I outlined before, where Hedgehog was discovered. OK. So it was a genetic screen in the flies, and these are genes that affect the embryonic development of the fly. And I'll show you this view of the fly embryo.
So this is an embryo here, and you see, it's an epithelial sheet surrounding the yolk. And what happens is, in early stages of development, a population of cells express the Twist gene. So that's Twist in the dark here. And this Twist gene causes these cells to basically invade into the middle of the embryo. So here are the Twist cells now, going into the inside of the embryo.
And then these cells undergo EMT, and they start to migrate around inside the embryo. What this is doing for the fly is that these are the cells that are going on to form the muscle. And if you look at your neighbor, you might notice that their muscles are on the inside of their body. So this process is basically putting these cells, by getting them to move, it's putting the cells in the right place for what they are going to differentiate into during embryonic development.
But this process, if activated during cancer, can allow these cells to be more mobile and to leave one organ and go into another organ. So cancer isn't inventing anything new here. It's corrupting a normal program that cells have and need during development, and it's inactivating it at an inappropriate time.
So these are all transcription factors. And what they do is, they repress the expression or function of cadherin such that the cells are no longer as sticky. So basically, when these transcription factors get turned on, it makes the cells less sticky to each other. And it also promotes their migration.
So it turns on genes that are important for migration. This process of EMT, as I mentioned, is reversible, and it also involves interactions between tumor and stromal cells. I'll show you an example of-- so in the fly embryo, one thing that's nice about the flies is, you can actually watch this happen live.
And so I'm going to show you a movie now, showing you the invasion process. We're going to have an embryo here. And what I'm going to show you is, we're looking at the cells that are expressing Twist and Snail, and they're on one side of the embryo. And I'm going to show you a section of the embryo that is analogous to this.
So when the cells move inside, they're going to disappear in this movie. So when you see the crease in the embryo, that's when the cells are invading into the middle of the embryo. And so the cell outlines are in magenta here. And what's labeled in green is a type of motor protein that's involved in the mobility of cells and, in this case, is involved in these cells moving into the interior of the embryo.
So here, you see the motor protein appearing, and that's when these cells are going to dive into the embryo. So it's almost like a waterfall. These cells are moving into the inside of the embryo through this process of invagination and, subsequently, EMT. There it goes again. You can see the cells are now on the inside.
So this process of EMT also involves interactions between the cancer cells and the stroma. And that's illustrated here because what you can see, what's being labeled here, you can see the nuclei of the cells, but these cells at the edge of the tumor are up-regulating this gene, which is an alpha-beta integrin, which I'll mention in just a minute.
And this is getting up-regulated right where the tumor contacts the stroma. And this gene, alpha-beta integrin, is a gene that's associated with motility and EMT. So on that slide here, one gene involved in motility is a class of genes called integrins.
And so this also results from the signaling between the tumor and the surrounding cells. And again, this is not something that cancer created. This is what happens during wound healing. So during wound healing, if you injure yourself, the wound recruits immune cells, and the immune cells signal to the surrounding skin or epithelial cells to undergo EMT in order to close and fill in the wound.
So this is a natural process that happens in your body that is simply getting corrupted by cancer cells. All right. So now let's talk about this last step of motility. So this is now how the cells would break out and move away.
Let's come back to this movie here. There are a few things I want you to notice about this movie. The first is, holy shit, that cell is moving. And so the first aspect of this is, because the cell is moving, it suggests that there's some sort of force being generated. So the cell is generating force.
And I'm not talking about like some type of mystical Jedi force. I'm talking about the mass times acceleration force, so like a physical force, OK? The second thing I want to point out about this movie is that you see that bacterium, and it's moving around, this cell is able to follow that bacterium. So the cell, in the force generation process, is very responsive to the signals that that cell is getting.
So the cell is generating force, and this force is responsive to signals. So for the remaining part of the lecture, I basically just want to tell you about how it is that the cell generates the force required to move itself around. And it involves a particular type of protein.
It's a component of the cytoskeleton, and we've talked about microtubules and the microtubule cytoskeleton and its role in segregating the chromosome. But there are other cytoskeletal systems that the cell has, and one is called the actin cytoskeleton, which is shown on the side above.
And actin, the actin cytoskeleton, is a system that is a biopolymer in the cell. So this is a biopolymer, like microtubules. And actin is a gene. And the actin gene encodes for a protein, the actin protein, and when the actin protein is made in the cell, it starts out as being just a single globular protein.
And when the actin is in this state, it is called globular or G-actin. But these subunits, these proteins, can come together and form a polymer. So they can go from being individual, isolated proteins to forming a polymer that forms a long skinny filament.
And this form of the protein that's forming a biopolymer is known as filamentous or F-actin. And these are long, skinny filaments. They can be hundreds of nanometers, even microns, in length, so hundreds of nanometers long. And the filament width is about 10 nanometers. So I'm just trying to give you a sense of dimensions, that this is a long, skinny filament that the cell can assemble.
And it assembles into these dense meshworks, which you can see in the slide up here. So this is the leading edge of the cell. So the cell would be migrating this way. And what you see in this cell is this densely branched network, and these are all actin filaments. So you get this huge dense forest of actin filaments that's right at the edge of the cell that is moving forward.
One thing I want to point out is, like all things in biology, this is not a one-way street. And so these biopolymers are very dynamic, meaning they can undergo assembly and disassembly, and they can do so on the timescale of seconds.
One last thing I want to point out about the actin filament is, there's a polarity to the filament such that there's an end, known as the plus end, where growth is favored, and there's an end, known as the minus end, where there's often de-polymerization.
So you can get a directional growth of the filament. So this is where growth happens. And so in this network that you're looking at, the way the actin is oriented-- so I'm going to draw just a cell here-- you have this dense meshwork of actin, and in this meshwork, there's a polarity to the way the actin filaments are oriented.
So the cell is migrating this way, and the plus ends of the actin filaments are facing out right at the surface. And the minus ends are back here. And so what you have, when the cell is migrating, is you have this biopolymer network that's growing on this end, but shrinking on the other end. And that allows the cell to generate a constant protrusive force. So it's the growth of actin filaments, the growth of F-actin, which generates a protrusive force.
Now if we consider the whole process of cell migration, what you can see is that initially, you're going to push the cell membrane forward. So you get a protrusion. It pushes it forward. That's often called a lamellipodium, or a pseudopod, and so this part of the cell moves forward.
But in order for the cell to have a net motion forward, it then has to stabilize that protrusion. And it stabilizes the protrusion by adhering to the substrate. So right now, we're just considering a cell moving on a flat substrate.
And so the way it attaches to the substrate is through cell matrix adhesion. And this cell matrix adhesion is mediated by another type of adhesion receptor known as an integrin. So the cell pushes forward, anchors itself on the substrate, pulls its body along, and then just repeats that cycle over and over again.
So the best way I can illustrate this is, if you think about it, you get your protrusion. You get your elbow out. You put down. You anchor. And it's just a repeated cycle, and it's able to migrate across the substrate. So it's kind of like a frontal toe mechanism.
You have cycles of protrusion. You generate traction, and then you pull, in order to translocate. And so that's how cells are migrating intuitively. This is just showing you that cells can pull on stuff. So not all cells migrate in this way. I just wanted to say that.
So 3D cells have other mechanisms to migrate that de-emphasize this traction mechanism that cells have. So you can get rid of all the integrins that a cell has, and they're still able to migrate. And that's because 2D emphasizes the role of adhesion, but if you can find the cell, then the cells are able to migrate.
So what's shown here are cells that are not confined. This is a confined cell in a micropipette. And you can see, it's the same type of cell, but the cell can migrate in confinement, but it can't migrate outside of confinement.
So in this sense, the cell is migrating through a different mode. And you can think of it as the cell is doing some type of chimney-ing maneuver. So it's able to get in a confined environment, push out against its surroundings, and that's how the cell then is able to generate traction in the absence of an integrin molecule. All right. Great. So we are set for now.