Flash and JavaScript are required for this feature.
Download the video from Internet Archive.
Description
Professor Martin talks about the regenerative and renewal capabilities of cells, covering adult stem cells and apoptosis. To help illustrate this, he uses the lining (epithelium) of the intestine as a model organ.
Instructor: Adam Martin
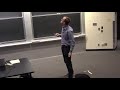
Lecture 24: Stem Cells, Apo...
ADAM MARTIN: All right. So in Monday's lecture, we talked about how cells replicate, OK? And today, I want to talk about how now an entire organ would essentially replicate. In this case, it's not going to divide, but it's going to regenerate or renew itself, OK?
And so this involves adult stem cells and also apoptosis , which you've heard a little bit about earlier in the course. And to explain this to you, I'm going to have basically a model organ that we'll use. And we'll use it for a couple lectures. And the model organ is going to be the lining of the intestine, OK?
So the lining of the intestine is an epithelial tissue. And I'll tell you a little bit about epithelia in just a minute. But you have the intestinal epithelium. And this is the-- these are the cells that are the lining of the intestine, OK? And one of the reasons that I've chosen this system is because the lining of your intestine has pretty remarkable regenerative capabilities, OK?
So your small intestine renews about every four to five days, OK? So the vast majority of your cells in the intestine were basically derived in the last four to five days, OK? So humans aren't as cool as some organisms, like newts, in that you can't cut off your arm and have it grow back. But at least we have the intestine, which undergoes a pretty dramatic regeneration, OK?
And this is not-- the intestine is unique in how rapid this is. But you have other tissues that also exhibit continual renewal, like your skin and your blood cells. And even the cells of your that line your insides of your lungs, they do exhibit renewal capabilities, OK? And so I'm going to use the intestine as a model system. It doesn't mean it doesn't happen in other tissues. But it just happens that we can-- we really understand the intestine system maybe a little bit more than many other systems. So I'm going to use it as an example.
So in thinking about the lining of your intestine, it's important, and it has important functions. One important function of this lining is it has to absorb nutrients from food going through your intestine, OK? So it exhibits a nutrient absorption function.
Now, the lining of your intimate intestine, much like your skin, is also a barrier between the inside of your body and the outside world, right? Because basically, the inside of your intestine is contiguous with the outside world, right? If you open your mouth, you can get down to the inside of your intestine, OK? So it serves also an important barrier function.
And one point I want to make about this system right now is that the intestinal epithelium, like many of your other organs, are composed of multiple cell types, OK? So it also has multiple cell types. OK. So let's now consider the lining of the intestine.
And the way the intestine morphologically looks is that there are a series of invaginations. So this is a cross-section view through the intestine. So you have to imagine that this is a cross-section view, but that this is a plane. And it's a plane with a lot of invaginations in the plane, but also protrusions out of the plane. And so you have to remember-- you have to think of this as a surface. And then it's wrapped up into a tube, OK?
So this is just a very simple cross-section image of the intestine, OK? And the lining of the intestine is a sheet of cells. So this lining is composed of many, many cells. They're columnar in morphology.
So what I've drawn here is just a small section of the intestine. This would be the lumen, out here. This is where the food is, or the food going through your intestine would be. And then below here, this is interstitial fluid inside of your body basically, interstitial, OK? So the food's up here. The rest of your body is down here.
And you can see that there are there there's a structure to it. It's not just a flat surface that's wrapped up, but there are invaginations. And the invaginations are known as intestinal crypts, right? Much like you would-- if you bury something, like a body, it would go below ground. So the crypt is below the surface of the lumen. And this these projections, out here, are known as villi. So they're the villi of your gut, OK?
Now, if we consider-- and this lining is made up of multiple cell types, which I've outlined up here and which are in your handout. So you don't have to write these down. But this is just making the point that there are various differentiated cell types. There are enterocytes, which are the absorptive cells. These are the cells that are taking in nutrients and transporting them into your body. There are enteroendocrine cells that play an important signaling function in the gut to regulate the biology of the gut system.
There are a goblet cells, which secrete mucus into the intestine system, which protects these epithelial cells from digestive enzymes that are present in the lumen. And there is this last cell type, the paneth cell, which functions as an important role in regulating stem cells, as I'll outline in just a minute. So these are all the differentiated cell types. But there's a type of cell that's an undifferentiated cell type. And that's the intestinal stem cell, which will be the hero of today's lecture.
All right. Now, if we consider just a small group of cells, what these cells look like is this, OK? So these are what are known as epithelial cells. And epithelial cells have certain properties. The first is you see that this end of the epithelial cell looks different from this end, OK? And this is called an apical-basal polarity.
So much like neurons have a polarity where the on one side of the neuron there are dendrites and on the other side of the neuron there's an axon, these cells have another type of polarity, which is called apical-basal polarity. So the side facing the lumen is apical. So the lumen would be up here. And the basal side would be down here, OK?
So this is basically oriented along this axis of the tissue, OK? where apical is on this side, basal is on this side, OK? And these projections from the individual cell, these are called microvilli. And essentially, these plasma membrane corrugations increase the surface area through which nutrients can be absorbed into these cells, OK?
Now, one other defining feature of these cells is they have proteins that protrude from the plasma membrane. And these are adhesion proteins that couple the cells together. And actually, the cells are stuck together much tighter than I'm drawing here, such that the cells form a barrier so that things can pass unregulated from the lumen into the body, OK? But these proteins, which I'm drawing sticking out of the membranes of the cells, are adhesion proteins.
And these adhesion proteins simply link the cells together such that they form a sheet of cells or tissue. So they link cells together, OK? So these are two of the key properties of epithelia. They have an apical-basal polarity. And they also exhibit cell-to-cell adhesion, such as the cells reach out and connect to each other. And they basically are glued together, or Velcroed together such that they don't easily come apart.
OK. Now, in considering this system, what I'm going to tell you is that there is renewal of this lining. And the renewal starts at the base of the crypts, OK? So there's going to be renewal. And this cell renewal is at the base of the crypts, right?
There are many of these crypts, right? You have you have like the surface, but there are many, many invaginations that are present in your gut. And the renewal is happening at the base of these crypts, OK? And that's because at the base of these crypts are where a type of cell, known as intestinal stem cells, lie, OK? So it's at the base of these crypts where there are what are known as intestinal stem cells.
And I'm going to abbreviate these ISCs for this, so I don't have to write out intestinal stem cell whenever I tell you about them, OK? Now, that's where renewal occurs. And if you just had more and more cells getting put in the system without any removal of cells, then the organ would get bigger and bigger, right? And so our intestine more or less staying the same size at this point in our life.
And so in addition to renewal, there's also cell death. And what happens when cell death occurs is that cells are shed from the intestinal lining into the lumen. So cells are shed into the lumen. And then they just go with the rest of the crap that's in your intestinal lumen. And it's eventually removed from the body, OK?
So some cells are leaving the tissue and going into the lumen after they've existed for a few days in the intestine, OK? So to have an organ then the constant size renewal has to essentially be more or less equal to death, OK? And so you can think of this as a type of homeostatic state for this tissue where, if renewal equals death, you have what is known as tissue homeostasis, where the number of cells in the system as a whole is basically remaining the same, even though there's constantly new cells going into this system. But then the cells are also-- the older cells are being removed from the system.
So what's really key in this process are these intestinal stem cells. So I'm first going to tell you about stem cells. And these are what I would define as adult stem cells, OK? So they're stem cells that are associated with a particular organ, OK? And I want to differentiate this right now between these types of stem cells and another type of stem cell that we're going to talk about later in the course, which is an embryonic stem cell.
So adult stem cells-- these stem cells, like intestinal stem cells, are associated with a specific organ. And they only give rise to cell types that are present in that organ, OK? So they're not-- they can't give rise to any type of cell type. Your adult stem cells are really specific-- organ-specific, we'll say.
In contrast, embryonic stem cells are-- these stem cells have more possibilities. They can give rise to pretty much any cell type in an entire organism, OK? So you can think of these adult stem cells as being more restricted in their fates than the embryonic stem cells. And so what adult stem cells are called is they're called multipotent, because they can give rise to multiple different cell types, but not all of the cell types that are present in an organism, right? An intestinal stem cell will not be giving rise to a blood cell or other cell types in other organs, right? It's restricted to just giving rise to cells that are present in that organ.
In contrast, embryonic stem cells are what are known as totipotent, or sometimes pluripotent, where this type of cell really is capable of making any type of cell that is present in an adult organism, OK? So that's less restricted than the adult stem cells. And we're going to come back to these embryonic stem cells towards the end of the course. But for understanding cancer and also tissue homeostasis in the intestine, we're really needing to focus on the adult stem cells.
OK. So these adult stem cells have two key properties. The first is what I just said. They're multipotent. And what multipotent means is that they can give rise to multiple different cell types. So this stem cell gives rise to multiple cell types. And this is associated usually with a single organ system.
OK. So in the case of the intestinal stem cell, the intestinal stem cell is multipotent. And it can give rise to many different terminally differentiated cells. And you can see here I've just written in example cell types that the intestinal cell could generate. It can generate any of the four different types of cells that I introduced to you at the beginning of the lecture.
The other key aspect of this system is that, in addition to generating all of these different cell types, the stem cell can also renew itself, OK? So the other key property is this self-renewal. So the intestinal stem cell also gives rise to another intestinal stem cell.
So it basically duplicates itself such that you still have a stem cell in the organ. And then one of the daughter cells is self-renewed and remains a stem cell. The other daughter cell can go on to divide further and give rise to these differentiated cell types, OK?
All right. So one question you might be asking yourself is, how is it that-- what regulates whether or not a cell will go on to differentiate or whether it will stay a stem cell? And the answer to this question involves communication between this stem cell and other cells in the system. And it involves a special type of cell called a stem cell niche cell.
And so I'm going to tell you about a model, which is the stem cell niche model. And what the stem cell niche model is, is that basically there is a niche or compartment which promotes the self-renewal of cells in that compartment that makes them stem cells. So the stem cell niche you can think of as a compartment where signals, similar to the types of signals that we've been talking about over the past four or five lectures, regulate the behavior of the cell to ensure self-renewal and to suppress differentiation, so a compartment where signals promote stem cell renewal.
I want to ask you one question before I move on, which is, how would you define-- how would you determine that there is a special type of cell that gives rise to all of the cells in an organ? If you were tasked with finding this and determining whether this was true or not, how might you do it? Does anyone have an idea of what experiment they would do, or what criteria they would have to determine whether or not this is a case?
Let's say I gave you a cell, right? In a dish. And I asked you to tell me whether or not you would think this is a stem cell or not. Yes, miles?
AUDIENCE: [INAUDIBLE]
ADAM MARTIN: Mm-hmm.
AUDIENCE: One cell is produced by the same cell, assuming that it's not a stem cell but if the cell [INAUDIBLE] it's a stem cell.
ADAM MARTIN: Mm-hmm. So Miles suggested he would like to take the cell that I just gifted him, and let it grow up, and determine whether it gives rise to multiple cell types. And Miles said that, if it just gave rise to a single cell type, that would suggest that it's not a stem cell. But if it gave rise to multiple cell types, then it could be a stem cell, OK? And it's this type of experiment that-- this type of experiment has been done, where you can take an intestine from mice, and even you can take tissue from humans, and you can associate the cells from each other so that you're left with single cells that are separate.
And you can then use some type of flow cytometry to separate cells. And you might be interested in a cell that maybe expresses some marker that you're interested in. And you can separate those cells and isolate them. And then you can take an individual cell and grow it in a dish, OK?
And this has been done for intestinal stem cells. And if you take an intestinal stem cell and you grow it in a dish, it can grow up and form a bulk of tissue. But what's really remarkable about the result of this experiment is that, from a stem cell, you get this massive tissue. But it self--organizes into a structure that very much resembles a normal gut, meaning that there are crypts where the stem cells are localized.
And then if you look at the different cell types in this, what's known as an organoid, you see all of the different cell types that are normally present in the gut, OK? And so this is an example of a type of experiment that's done to show whether or not a certain cell has the capability of functioning like a stem cell, OK? So if you start with a stem cell, you can regenerate the entire organ system essentially.
You might also be familiar with bone marrow transplants. And so if you kill all the hematopoietic stem cells in, let's say, a mouse, then you can transplant in a single hematopoietic stem cell into that system. And it will regenerate all the blood cells in that system, OK? So those are just a few examples of how one might functionally define a stem cell in an experimental setting.
All right. So now, I want to tell you about the types of signals and how these signals are promoting stem cell renewal, OK? So in this case, the niche, stem cell niche is going to be right here. And the types of signals involved-- I'll just draw some cells here. One of the types of cells that's part of the niche in the intestine is the cell type that I introduced you as the paneth cell.
And these paneth cells localized to the base of the crypts together with the intestinal stem cells. And what paneth cells do is they send a signal to neighboring cells. And this signal is a Wnt signal, which I'll tell you about in just a minute. And so this is the intestinal stem cell here. And the paneth cell is signaling to that intestinal stem cell through a secreted ligand known as Wnt. So Wnt is a secreted ligand.
And this signal is what's known as a juxtacrine signal, which just means that the cells have to be adjacent, or juxtaposed, to each other in order for the sending cell to signal to its neighbor, OK? So it's not signaling long range, but it's signaling to a neighboring cell, OK?
So now, if we think about this intestinal stem cell, one thing intestinal stem cells do as they divide, right? So this intestinal stem cell could divide. Here is a cell in mitosis. It rounds up and divides. Here, you still have your paneth cell.
And then when this cell divides, then it's going to be two cells, OK? So now, paneth cell, another cell. Here the two daughter cells. Here's the paneth cell. The paneth cell is still secreting this Wnt signal. So you have Wnt getting secreted. And it's going to signal to its neighbor. But this cell is starting to get farther and farther away from that signal, right? So you can imagine this is happening right here in the tissue, where this cell is at the boundary of the niche, OK?
So this cell that gets pushed out of the niche is going to start to differentiate, OK? Because it's no longer seeing the signal, OK? So it's the lack of the Wnt signal which tells cells basically that they should start differentiating into the various cell types of the gut. But the cells that are stuck at the base of the crypt, down with the paneth cells, are still getting the Wnt signal. And so they remain an intestinal stem cell, OK?
So here is just a diagram showing you part of that. So the niche is down here, where you have the stem cells. The paneth cells would be some of these blue cells at the base. But there are also other cells below the epithelial lining known as stromal cells that are also secreting Wnt. And so this compartment down here has high Wnt ligan activity. And that tells cells that remain here to stay stem cells. But the cells that are getting pushed up and moving up towards the lumen, they no longer receive this signal. And so they are going to start to differentiate, OK?
So you can think of this system as almost a conveyor belt. There's a conveyor belt-like movement of cells from the base of the crypt up towards the tip of the villis, OK? And when the cells move away from the niche cells, then they no longer have the self-renewal signal. And therefore, they go on to differentiate.
OK. We'll go back for now. All right. Now, I want to tell you a little bit about this signal, Wnt, because it's something that's come up before in the lecture, even though you might not know it. So Wnt is a ligand. So it functions much like a growth factor. This is a protein that is secreted from the cell, and then binds to receptors on other cells, and induces signaling events in those cells, OK?
And Wnt stands for-- the W stands for wingless, OK? So you remember from earlier in the semester, wingless was identified as a mutant that disrupts the formation of wings in the fly, OK? So one of the places where these genes were discovered is in the fly. The nt of Wnt comes from int 1, which stands for the integration of mouse mammary virus 1. Sorry, that's a little bit more of a mouthful. The integration of mouse-- actually, sorry, mouse mammary tumor virus 1. I forgot the tumor part of it.
OK. So the W in Wnt is from wingless. The nt in Wnt is from int 1, OK? And so as you can see, there are two different systems where this type of gene was discovered. They are very disparate from each other. One was in a developmental mutant, in the fruit fly. The other was in a mouse system, where the integration of the virus caused over-expression of Wnt, which caused it as tumor genesis, OK?
So these disparate systems led to the identification of this Wnt molecule. And this is a defining member of a signaling pathway that regulates stem cell renewal. And I just want to briefly go through the logic of the signaling pathway, because I want you to get a sense that not all signaling pathways are like Ras-MAP kinase, but there can be different regulatory logic, OK?
So what's the regulatory logic of this pathway? So the regulatory logic is shown in this cartoon. And I'm going to start with a cell that does not see Wnt ligand. So that would be the case on the left here. So if there's no Wnt ligand-- I want you to focus on what's going on here-- there's a complex that's present in the cytoplasm of the cell. And what it's doing is it's destroying this beta-catenin protein, OK?
So what beta-catenin is-- among other things, it's a transcriptional coactivator. So it's basically a transcription factor. So it works with another protein to regulate the expression of certain genes, OK? And in the absence of Wnt, this beta-catenin transcription factor is destroyed and is not able to get into the nucleus, OK?
So if there's no Wnt, then beta-catenin is destroyed. And it's destroyed using a system that I introduced in Monday's lecture, which is regulated proteolysis by polyubiquitination, OK? So the first real-- so you have regulated proteolysis by polyubiquitination.
So the way this works, as seen here, is that beta-catenin is bound by this complex. And there's a kinase that phosphorylates beta-catenin. And then the phosphorylated beta-catenin recruits this E3 ubiquitin ligase, which polyubiquitinates it, OK? So that leads to the destruction of beta-catenin in the absence of a Wnt signal.
But when Wnt ligand is around, this leads to the disassembly of this complex, which is known as the destruction complex. And that leads to beta-catenin accumulating. And once it accumulates, it goes into the nucleus and starts changing gene expression, OK?
So in the presence of Wnt, beta-catenin is nuclear. And that's where it needs to be, if it's going to regulate gene expression, OK? So what you see is the logic of this pathway is you have a double negative, where you have a complex, which is known as the destruction complex. And it includes this gene, APC, which we're going to talk about on Friday. This destruction complex is inhibiting beta-catenin by destroying it.
And the way that Wnt induces beta-catenin activation is by inhibiting the inhibitor. So Wnt inhibits the destruction complex, which then stabilizes beta-catenin and allows it to go to the nucleus, OK? So the other piece of the logic here is you have inhibition of an inhibitor to activate beta-catenin.
Any questions on the pathway? OK. So now that we have our intestinal stem cells and we have a way, by increasing Wnt in this compartment, to maintain the intestinal stem cells through self-renewal, now we have to talk about the compensatory mechanism of death, which allows this tissue to maintain homeostasis. OK. So death-- in this case, death is going to be useful. And the process of death is called apoptosis. And apoptosis is Greek for falling off.
And that's essentially what these cells are doing, because the cells, as they move from the base of the crypt up towards the lumen-- eventually, they're going to fall off into the lumen of the intestine. So again, I'll draw. Here's a villus. This is now a villus.
And so what happens is, at the tip of the villus, cells are going to be shed from the lining of the epithelium into the lumen. The lumen is up here. This is the villus. And the cells are going to shed off into the lumen and be removed from the organ system, OK? So cells are shed into the lumen here, OK? And this is going to balance the renewal at the base of the crypts such that there's homeostasis.
So I just-- the movie I was showing you at the beginning of class was a movie showing you what happens to a cell undergoing apoptosis. So in this case, the cell is binucleate and unhappy. And then you're going to see that it basically explodes, OK?
So that's a cell undergoing apoptosis. But you see that there is a clear change in cell morphology and physiology associated with this. And I just want to point out that this is also something that we talked about earlier in the course. And we talked about experiments, a genetic screen that led to the identification of the pathway that regulates apoptosis.
And that was done by Robert Horvitz. Much of it was done by Robert Horvitz in his lab here at MIT. And for that work, Robert Horvitz, in addition to his colleagues, won the 2002 Nobel Prize. So you'll recall this is something we talked about in the context of a genetic screen. But this is something that's-- this is what it's doing in your intestine system. It's balancing renewal so that you have homeostasis.
So during apoptosis, a cell goes through a series of changes. First-- or what happens eventually is the nucleus becomes fragmented and chromosomal DNA even gets fragmented. It gets chopped up. And also, the plasma membrane also starts to bleb and fragment such that it breaks up into what are known as these apoptotic bodies, OK?
And so you can think of these apoptotic bodies as bite-sized pieces of cell that neighboring phagocytic cells can eat up and remove them from the body, OK? So in this case, the cells are being shed into the lumen. So they don't need to get eaten up, because they're just going to go out of the digestive tract.
So there are-- cells have numerous ways to activate this apoptosis process. I'm going to tell you about two types of signals that regulate whether or not a cell undergoes apoptosis. The first is that there can be a signal that basically tells the cell to kill itself, OK? So you can think of this as a kill signal.
And one type of way to activate this signal is if the DNA is irreparably damaged. So if there is a high level of DNA damage, this induces a signaling process in the cell. And one of the results of that signal-- in addition to regulating the cell cycle, if the DNA damage is great enough, it will induce an apoptotic signal. And it will activate the pathway that the Horvitz lab elucidated in the worm, OK? So there's a signal. And that leads to apoptosis and death.
Another type of signal that's critically important for tissue homeostasis and determining whether or not cells live or die is a survival signal. So there are cell survival signals. And many of the growth factors, such as EGF which you've heard about-- in addition to inducing proliferation, these growth factors also tell the cell not to die, OK? So these could be growth factors.
And what these cell survival signals do is they repress apoptosis. And so you can think of it where you have a cell constantly needs to be communicated to. And it needs to be told don't die, don't die, don't die, don't die. And then if you remove that signal, it won't be getting that information anymore. And it can undergo apoptosis, OK?
So if we were to remove this signal, you remove the brakes on apoptosis. And the cell will undergo cell death, OK? So this ensures that you don't have a cell just kind of like going on and doing its own thing, because cells, in order to live, often need to have some sort of signal from another cell that tells them to live. And so there's some coordination between cells such that you don't have cells rampantly dividing out of control.
Now, the reason we're doing this right before we talk about cancer on Friday is because everything that I'm telling you is really essential to understand how a tumor is formed in an organ system, OK? And I want to end the lecture by just planting a seed of an idea in your heads before we move on to talk about cancer on Friday. And I want you to think about the organization of this system, where you have stem cells undergoing renewal.
And then the stem cells are just a small fraction of the cells in the system. And they're dividing slowly, OK? So let's think about the stem cells. The DNA on the stem cells, these are dividing slowly. And because they're dividing slowly, they're not going to-- their DNA is not going to accumulate as many mutations. So there is going to be fewer mutations.
But these are the cells, and this is the genomic DNA that is going to stay with the organ, OK? So the stem cells are like the crown jewels of the organ. This is the material the organ wants to protect, because it's what's going to be lasting in the organ the entire lifetime of the organism, OK?
So you get slow division here and self-renewal. And this cell will stay with the organ. But then where most of the mitosis and cell division happens and replication happens, it leads to an expansion of cells that all differentiate. And because the cells all differentiate, they will all eventually die and get removed from the organ, OK? And this is termed transient amplification.
So when there's transient amplification of one of the daughters of this stem cell, this is where there's rapid division. And where there is rapid replication and division, this is where you can get the most mutations. But from the standpoint of cancer, that doesn't really matter, right? Because in order to have a tumor, the cells have to stay in the body. And so all of these cells are going to undergo programmed cell death, and then be shed into the lumen of the intestine and removed from the organism entirely, OK?
And so this is actually one important way that our organs and our bodies prevent tumors from happening, because the cell type that is going to remain in our body is the one that's protected from accumulating mutations, OK? And we'll come back to this on Friday. And so I will see you on Friday. And we'll talk about cancer on Friday.