Flash and JavaScript are required for this feature.
Download the video from Internet Archive.
Description
Continuing the topic of immunity, Professor Martin talks about how immune cells are able to see within a cell in order to address an infection in the body. He focuses on the processes of antigen presentation, isotype switching, and autoimmunity.
Instructor: Adam Martin
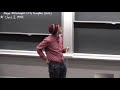
Lecture 31: Immunology 2—...
PROFESSOR: All right. So today, we're going to talk about immunity again. And so this movie up on the screen here-- this is a cell. You can see the outline of the cells kind of around here. That's the outline of the cell.
But what you can see is that there is something in the cell moving around, and that is an intracellular bacteria called listeria. And you can see it's rocketing around in this cell. It's having a total party in this cell, and what you'll see here is you can often see the bacteria push out from the cell.
So if you look here, one is going to push right now. See? There it goes. And it kind of runs into the edge of the cell and pushes out, and this enables the bacteria to spread from cell to cell without actually going into the extracellular space surrounding the cells, OK?
So let's take a hypothetical situation. So listeria is a foodborne illness. It causes a nasty sort of intestinal disease. So Brett, do you want these bacteria having a party in your cells?
AUDIENCE: Unlikely.
PROFESSOR: Hell no. OK, Malik, do you want these bacteria having a party in your cells? Hell no. Carmen, do you want these bacteria having a party in your cells?
AUDIENCE: Hell no.
PROFESSOR: Hell no! Yes. OK, so our body has to have some way to sort of address this type of an illness, and the problem is if you're thinking about what we discussed on Wednesday, is-- all right. So you're hosting this party, right? This is your cell. So you have a host cell-- that's your cell-- and you have an intracellular pathogen, such as a bacteria or it could also be a virus, and they're essentially using your generous host cell to reproduce itself to spread to other cells of the body.
And so you don't want that, but the problem is that-- I told you about B cells, so remember B cells-- they have an antigen receptor. It's initially on their plasma membrane. It can also be secreted, and it's secreted into the extracellular space. The problem is that these pathogens are inside the cell, and there's a plasma membrane separating them from the antigen receptors that you need to recognize them, OK? So this presents an issue.
It's also the case for T cells, because as you heard on Wednesday, T cells only have this membrane-bound form of the receptor, and the antigen recognition domains of all of these are extracellular, so there is really-- with just this system, there's no way for your immune cells to see in the cells. So today, I want to talk about how is it that the immune cells are able-- how our immune cells are able to see within the cell in order to address an infection like this one, with listeria. OK?
And the first part of the answer is that it involves a process known as antigen presentation. And antigen presentation is the process by which peptides, so short sequences of amino acids, are presented and displayed on the surface of the cell for the immune system-- for immune cells to see. So here, peptides are displayed on the cell surface for immune cells to see them. And in this specific case, it's going to be for the T cells to observe what's going on inside the cell, OK?
So this mechanism involves another molecule, which I briefly introduced. It's called the major histocompatibility complex, which is abbreviated MHC. So when I referred to MHC in Wednesday's lecture, I was referring to this major histocompatibility complex. And there are two classes of MHCs. Thankfully, the first one is known as class 1, so class 1 MHC, and class 1 MHC looks like this.
Like many of the immune receptors that I've talked about, it has a heavy chain, which is this long polypeptide light blue, and it has a light chain in purple. So the MHC is composed of these two separate polypeptides. They're encoded by different genes, and then it assembles into this structure shown here. So this molecule has two Ig domains, and these are proximal to the plasma membrane.
And this thing is all inserted in the plasma membrane. It's an integral membrane protein. And then distal to the plasma membrane is this structure here, and if you look at the crystal structure, it's kind of like a sheet-- a beta sheet with two alpha helices. And altogether what it does is it basically creates, like, a little cup, OK? So it's creating, like, a cup.
And what sits in this cup is a peptide, so you get peptides, and the peptides sort of sit in that hand, if you will. And some of the amino acids from that peptide are sticking out and they're sort of displayed away from the MHC molecule. So this is basically a hand that holds peptides and displays them on the outside of the cell, right? So the outside of the cell here is up. This would be the exoplasm out here, and it's displaying these peptides for immune cells like T cells to observe.
All right. So class 1 MHC is a class that's expressed on all nucleated cells in your body. So that's all of your nucleotide cells are synthesizing in a class 1 MHC, and then it's sort of being displayed on the surface. And the peptides that are held by this class 1 MHC-- the peptides here are being derived from a specific place in the cell, which is the cytoplasm. So the peptides are from the cytoplasm, so this is the source of the peptides, and I'll tell you how these peptides are sort of loaded on to this MHC molecule.
So the MHC molecule is a membrane protein, so it's translated on the endoplasmic reticulum, and its extracellular domain is initially present in the lumen of the ER. And the peptides are from proteins that are present in the cytoplasm, and what happens to these proteins-- and this occurs for unfolded proteins, but also for proteins that might be ubiquitinated-- is that they're processed by the proteasome, which is this kind of a shredder-like function for proteins, and it cuts up the proteins into little snippets, or peptides, that can then be pumped into the lumen of the ER through this transporter, TAP. So these peptides can be taken and transported into the lumen of the ER, and that's where they're loaded onto the class 1 MHC molecule.
But the source peptides is from proteins that are in the cytoplasm. They're processed by the proteasome. So then, once you have a peptide-MHC complex, it can then be trafficked through the normal vesicle trafficking pathway all the way out to the plasma membrane of the cell where now that peptide will be displayed for T cells to observe.
And so the peptides here, they're processed by the proteasome-- processed or cut by the proteasome-- and then the type of T cell that's going to look at these class 1 molecules-- they are known as seeds CD8 positive T cells. So this is the first class of MHC molecule. Because there is a class 1, that means there also must be a class 2, which there is. And so class 2 MHCs are fundamentally different in all of these properties. The function shared by these MHC molecules is they both display peptides on the surface of the cell. So MHC molecules do display peptides on the surface, which is known as antigen presentation.
But other than that, MHC class 2 is pretty different. You see the structure of MHC class 2 is very similar to that of class 1, but you see that rather than having a heavy and a light chain, here there are two chains that are roughly of equal size. And so these are encoded by different genes than the class 1 molecule, and they encode different proteins.
But the overall structural similarity is very similar, so there are two Ig domains, they're proximal to the plasma membrane, and then there's this structure at the very end of the MHC molecule, which has this groove in it which can hold a peptide that would be displayed on the surface of the cell. And there, you see the groove and you see the peptide that is present in it.
All right, so one big difference between class 1 and class 2 is that class 2 is expressed on a much more restricted set of cells. So class 2 MHCs are expressed specifically on specialized cells known as antigen-presenting cells, and these antigen presenting cells include cells like B cells, which are the ones that I'll focus on, but also phagocytic that can phagocytose foreign substances-- phagocytic cells-- and there's another cell type called the dendritic cell, which is also an antigen-presenting cell. I'm going to focus on the B cells. So class 1 is expressed everywhere. Class 2 is really expressed on these professional antigen-presenting cells.
And the way that the peptides-- the source of the peptides and the way they're generated is also very different. So peptides for class 2 come from the extracellular space, and they are processed by lysosomal proteases. And so I'll show you how that looks in cartoon form.
So for MHC class 2, the peptides are from the extracellular space. And so we've talked about ways that cells can take in material. One way is through endocytosis, right? So if this is my antigen, the antigen could be endocytosed by the cell, and now it's in a vesicle that's present in the cell. And so if you endocytose this protein, then it's now in a vesicle, and one compartment that it can go to is the lysosome, where are these there are these lysosomal proteases they can then chop up this protein into little snippets, or peptides.
And so MHC class 2, again, is translated at the end of endoplasmic reticulum, like all plasma membrane proteins. But in the endoplasmic reticulum, you see the peptide groove is blocked such that peptides derived from the cytoplasm can't interact with class 2, but then is trafficked to a unique compartment which can combine with the compartment that has the peptides that originated from outside the cell. And then those can get loaded onto this class 2 MHC molecule, and then this can be recognized by T cells. But in this case, it is a-- oh, I endocytosed my chalk. I need to get it back. Here. So in this case, it's not a CD8 T cell that's recognizing it, but a CD4 positive T cell.
OK, so let me briefly review what I just went through, and review the differences between class 1 and class 2. So class 1 MHC is expressed on all nucleated cells, whereas class 2 is much more restricted, being expressed specifically on antigen-presenting cells. The T cells that recognize these two classes are different. Class 1's recognized by CD8 positive T cells. Class 2 is recognized by CD4 positive T cells.
And the source of the antigen is different in these two cases. The source of the antigen for class 1 is the cytoplasm. For class 2, it's the extracellular space. So the different MHCs are sampling different sort of pools of proteins. And where the peptide is loaded is distinct between these two, which allows these distinct classes to basically discriminate between the sources of the peptides that they're loading. So for class 1, that's in the ER. For class 2, it arises from a vesicle compartment that results from endocytosis of an antigen from outside the cell.
All right. Now, the type of molecule that recognizes this MHC peptide complex is the T cell receptor, which I briefly outlined on Wednesday, but now we're going to talk about it in much more detail. So the T cell receptor, or TCR-- and I talked about its structure which is shown up on the slide, but I'll just draw more simply here. If this is the plasma membrane, this is the cytoplasm, and this is the exoplasm facing down, then this T cell receptor has two chains. One is called the alpha chain, and the second is called the beta chain. And each is comprised of two Ig domains, which you see up there.
So the T cell receptor here is in pink. You can see an Ig domain there on one strand-- Ig domain there. And you have another two Ig domains on the other subunit, and this receptor, the T cell receptor, recognizes antigens through its variable domain, which is here. And it's binding basically to the end of this receptor, so this is a sort of ribbon diagram of a structure for a T cell receptor.
The plasma membrane would be up here. This is the end of the T cell receptor. And MHC is in green, and it's holding a peptide here in yellow. And you can see how the TCR is sort of interacting or docking to this MHC-peptide complex.
So for the T cell receptor to interact and bind to MHC, you have to have a T cell receptor that recognizes the specific conformation of the peptide that is being sort of extended away from the cell. So let's say this is my T cell receptor, and I'm going around and searching for cells that might want to look at this. Then if I had a T cell receptor that was like this, it's not going to be able to stick to this MHC-peptide complex.
However, if I had a T cell receptor that had the right conformation, because there are different types of T cell receptors, it might be able to dock on and stick to the peptide, and then the T cell is now stuck to the peptide-MHC complex, OK? So there are different T cell receptors. There's a diversity of T cell receptors, and they're able to discriminate between different peptides loaded onto MHC.
OK, so now, we have to think about where this diversity of T cell receptors comes from. There's a diversity of TCRs, and lucky for you, the mechanism that generates the diversity of TCRs is the same that generates diversity for antibodies. Now, Georgia asked a really good and really important question at the end of lecture on Wednesday, which is-- she asked if this sort of rearrangement of gene segments in the variable domain of the antibody was due to splicing or recombination at the genomic locus. And the answer is that it's recombination at the genomic locus, and that's a very important point.
So here's a diagram for the beta chain of the TCR. You can see that like the B cell receptor, there's a gene rearrangement in the genomic DNA that brings V, D, and J segments together to make the variable chain of the T cell receptor. So like the B cell receptor, there is a gene rearrangement, also known as VDJ recombination, and this is not splicing of the transcript. This is in the genomic DNA-- a very important point, because by having this happen in the genomic DNA, it creates an irreversible change in that genomic DNA such that all subsequent cells that are derived from that original B or T cell are going to express the identical B or T cell receptor. So it's not splicing, but it's a real sort of irreversible change to the genomic DNA.
So you have a diversity of T cell receptors, but the T cell receptor is not the only thing that enables the T cell to interact with whatever cell is presenting the antigen. There are these other co-receptors which are important. So there are co-receptors on the T cell-- this is on the T cell-- and the co-receptors are CD4 and CD8, and they're expressed on different subsets of T cells.
And these co-receptors-- because it's not sufficient for just the T cell receptor to interact with a specific peptide, it also requires this co-receptor in order to get an immune response. So the logic is that if the T cell receptor and the co-receptor both bind to the MHC, then you get a particular type of response, so you need both. And CD4 cells recognize the class 2 of MHC. CD8 recognizes class 1 MHC.
So you have these two different subsets of T cells and they recognize these distinct MHC complexes. So my question for you is what should these CD8 positive T cells do? To help with that, you might want to look at where the peptides are coming from that are presented on the class 1 MHCs, which are going to be presenting specifically to CD8. So what should these do?
What does it mean if you have a class 1 MHC molecule containing a peptide that looks foreign? Well, where do the peptides come from? What's that, Patricia? Patricia is right. They're coming from the cytosol.
So if you have foreign elements coming from the cytosol, what might that mean for that cell? Good, bad, irrelevant? What's that?
AUDIENCE: [INAUDIBLE]
PROFESSOR: What's that? OK, Brett's saying it needs to be dealt with, and I totally agree. Here's one scenario-- would be the scenario I showed you in the beginning of class where you have some sort of intracellular parasite that is basically using the host cell for its own evil purposes to produce more viruses or more bacteria. So if the immune cell has some sort of indication that this is going wrong, another example is in cancer, because if you have oncogenic mutations in certain genes, then those could be recognized as foreign. And so an appropriate response might be to do something to that cell that would limit the expansion of the tumor.
Or in the case of an intracellular parasite, you really need to terminate the cell so that you stem the tide of viruses that are going to be produced by that cell. So the response should be to kill. So it was CD8 positive. If you have a CD8 positive T cell, it indicates there's something wrong inside that cell, and the response should be to kill it. And these CD8 positive T cells are known as killer or cytotoxic T cells.
So what happens if a CD8 positive T cell recognizes a MHC class 1 peptide complex, then it releases materials from inside it that perforate that cell and lead it to undergo cell death. So it's a way of limiting an infection by killing the cells that the virus or pathogen is using to reproduce itself. OK, what about CD4 positive? What should be the response of a CD4 positive T cell? Should it also kill? Should be like the T-1000? No one gets my cultural references. Yeah. Should it be the Terminator 2? No. Yes or no? Who thinks it should terminate? OK. Steven, can you tell us your logic? Why should it not terminate?
AUDIENCE: Because it's a [INAUDIBLE] B cell from the same [INAUDIBLE].
PROFESSOR: What are the MHC class 2 cells?
AUDIENCE: Like, a B cell or [INAUDIBLE].
PROFESSOR: Yeah. It's not only a B cell, it's a B cell that recognizes the foreign agent that you're infected with. Yeah, Brett?
AUDIENCE: So those B cells are antigen presenting cells. They have the information about what is bad or what is wrong in probably other cells? So like, oh, hey, we have this information. You should go and mobilize.
PROFESSOR: They're binding something that it recognizes as foreign, internalizing it, and then presenting bits of that foreign element on the outside of itself.
AUDIENCE: Shoots the messenger.
PROFESSOR: What's that? It's shooting the messenger, exactly. Yeah. So it would be an extremely bad idea for the CD4 positive T cell to kill what's presenting the antigen, because you would kill the exact cell that you would need to fight that antigen, right? Here you have a B cell. It would be a B cell that's producing an antibody that actually can produce antibodies that might be able to neutralize that foreign invader, and so you don't want to kill it. You want to help it or enhance the B cell function.
And so these CD4 positive cells are known as helper T cells, and they enhance B cell function in a number of different ways. Oh, I should point out where this happens. So this sort of interaction between B and T cells happens in the lymph node, because in the lymph node, you have antigen-presenting cells, or even soluble antigens, coming into these lymph nodes. And you also have B and T cells, and this is kind of like the B and T cell hangout to get sort of, like, interactions between these two distinct immune cell types.
And when you get sort of a B cell that presents an antigen that's recognized by a T cell, then the T cell enhances B cell function, and it does so in a number of different ways. The first way that it induces a response in the B cell, known as affinity maturation. And this affinity maturation results from a hypermutation of the variable domain of the antibody such that you get even more diversity, and such that a B cell can be selected that even has a tighter binding to the antigen.
So for affinity maturation, this is responsible for the transition in binding from a more weak binding to a tighter binding, which I talked about as being a difference between the primary infection and the secondary sort of immune response, OK? So the antibodies get better because of this B and T cell interaction and this affinity maturation process. One other thing that happens is that the B cells can produce different classes or isotypes of antibodies, and this is known as isotype switching.
And so this is, again, the genomic locus for the heavy chain of an immunoglobulin. You see, here's the VDJ segment, so it's undergone VDJ recombination, and then what you see are these different blue regions here. Each of these are exons that encode a different isotope for the antibody. So the first one is mu, and so that produces IgM when that's the one that's proximal to VDJ. So if you have IgM, that's the initial state of the antibody, and that's initially membrane bound and serves as the B cell receptor.
But each of these different constant domains, even though they're not undergoing variation, they have different effector functions and can do different things for the body. So for example, if you had isotype switching and you had a recombination event that brought this gamma 2 segment together with VDJ, that would produce the isotype which is known as IgG, and IgG is a highly secreted form of the antibody that is highly effective for bacterial infections because it's secreted in the blood, and it's able to neutralize bacteria and limit the infection that way. But there are other possibilities, because you have all of these different possibilities.
And so you could get VDJ together with this alpha, and that would produce an isotype known as IgA. And IgA promotes mucosal immunity because it's able to pass through the epithelial linings. In addition, IgE is another type of antibody, and the constant domains are constant for each of isotypes, but they recruit different effector functions.
So IgG would be hitting bacteria by promoting phagocytosis of those bacteria. IgE, in contrast, is especially good at dealing with worms, right? So if you have an intracellular-- or not intracellular, but like, an intestinal worm or something like that, then IgE-- its effector functions are better at dealing with that. So this process of isotype switching sort of allows the immune system to adapt to tackle a particular type of pathogen.
All right. The last way in which T cells enhance this function is by promoting the differentiation of B cells into different types of B cells. One of those types of B cells is known as a memory B cell, and the memory B cell is a B cell that can last in the body for decades, even if the antigen is not present. So this mediates sort of the memory of the immune system.
And so just to summarize what I just told you, if you have a B cell and it recognizes an antigen, which could be a protein, it would internalize that protein via endocytosis and then process it so that it can display peptides from that antigen on its surface. And if that's recognized by a T cell, then that leads to an interaction between the T and B cell that will lead to these different things happening, such as affinity maturation, isotype switching, so the red here would be a different constant chain on this same variable chain. So the variable chain doesn't change with the isotype switching, so it's still always able to recognize that antigen-- it's just recruiting different effector functions.
And you can also get differentiation of B cells into plasma cells, which really secrete a ton of antibody, and therefore help the body fight infection. Now this is important because for a vaccine to be effective, you need to engage this T cell response such that you have all of these things happening. So all of these things need to happen for an effective vaccine.
So for an effective vaccine, you can't just activate the humoral side of the immune system. You have to activate both the humoral and the cell-mediated sides such that they interact in order to enhance the immune response. All right.
Now I'm going to move on and talk about a big problem that the immune system has, which is that it needs to somehow be able to discriminate between self and foreign, right? And so if you have your immune system recognizing an antigen that is natively part of your body, that results in an autoimmune disease. So there's a balance in the immune system between tolerating antigens or attacking them, and if it's attacking a native antigen, then it's autoimmune.
And this is a huge problem because, if you think about it, because we've talked about the B cell receptor, the antibody, and the T cell receptor, right? Our bodies are generating tens of millions of these receptors that are diverse and can recognize different molecules. So our body is generating tens of millions of antigen receptors, and it does this constitutively, so that means that it's just doing it automatically. You don't even need to be infected for this to happen. This is just part of the development of B and T cells. OK, so it's constitutive, doesn't require infection-- constitutive.
In addition, it's totally random. Your body could generate any sort of combination of V, Ds, and Js, and it could mutate in a certain way that it's likely that at some point during your lifetime you're going to generate a receptor that recognizes a native protein in your body. So it's totally random-- at least what the sort of rearrangement of VDJ gives. That process is constitutive and random.
So I just want to point out several diseases that are caused by autoimmunity, and I've distinguished them based on whether the disease involves the generation of antibodies that recognize self or T cells that recognize self. So for antibodies, there's a disease, myasthenia gravis, which an individual's-- individuals generate an antibody against a receptor for a neurotransmitter, acetylcholine. And acetylcholine is the neurotransmitter which is predominantly involved in sending signals from a motor neuron to a muscle, and therefore antibodies that inhibit this receptor result in muscle weakness.
Now self antibodies can also result in diabetes, and individuals can develop antibodies that recognize and inhibit the insulin receptor, and this leads to insulin resistance and diabetes mellitus. Some examples of T cell mediated diseases are-- if you recall back in the beginning of the month, when we talked about electrical signaling in neurons, I told you about the myelin sheath and how this increases the speed of the action potential along that axon. And if T cells attack the myelin sheath, then it disrupts this process of electrical signaling, and that results in a devastating disease, which is multiple sclerosis.
Autoimmune disease involving T cells also involves diabetes, and if T cells attack and destroy the islet cells of the pancreas, this also disrupts the body's ability to produce insulin, and that results in type 1 diabetes. So I'm sure many of you know people with these types of diseases, and they're obviously of significant impact both in this country and around the world.
So the problem for the cells in our body and the immune system is that the immune system has to have some sort of way to distinguish between self and foreign. So how is it that the immune system does this? And also, it has to have different responses to self-recognition versus foreign recognition. So what should the immune system's response be if there is a self recognition? What should it do to the cells that recognizes a native protein? Rachel?
AUDIENCE: [INAUDIBLE]
PROFESSOR: It could delete that cell. What Rachel said is you should get rid of it. And so one way to think about this process is there's a bit of a Darwinian natural selection going on in the body, and if there is a self recognition, then there should be a negative selection against that cell, so there should be negative selection. This cell should be more unfit, whereas if it's obviously foreign, then there should be positive selection. This B cell should be more fit.
And what Rachel suggested is to get rid of the cell, which is a great idea, because if you kill off the cell then you won't generate any more cells that have that recognition against self. So negative selection is mediated by apoptosis and cell death. And positive selection could be both the activation of the cell and also its proliferation. As you see up on the slide there, that orange cell-- if it was recognizing a foreign antigen, would get activated and it would undergo a monoclonal expansion. All the cells resulting from that expansion would express the same antibody and therefore recognize that antigen, so this would result in cell division or expansion of that population of cell.
So now we know what to do with self versus foreign, but how is it that we distinguish between self and foreign? So how does the immune system distinguish self from foreign? And there are several mechanisms to do this. The first is that the organs-- the lymphoid organs-- where are these B and T cells mature and undergo these genomic rearrangements are largely protected from foreign agents. So there are basically only self antigens in the generative lymphoid organs. These are the lymphoid organs were B and T cells are being generated.
So the generative lymphoid organs would be the bone marrow for B cells and the thymus for T cells. Therefore, if a B or T cell-- if its receptor engages with something very tightly during its development, that's a signal for the immune system to delete and kill off that cell. So if you get self recognition here, you get apoptosis and deletion of that cell.
The second way that the body is able to distinguish is that it responds to antigens specifically when there is an innate immune response, or if it responds better when there is an innate immune response. So you can think of it like a coincidence detector, right? If you have an immune cell and it recognizes an antigen, and there's also an innate immune response, that's a strong indication that this is foreign. So this would indicate "foreign" to the immune system.
If there is antigen only and the body is not mounting an innate immune response, it's much more likely that this will generate a robust immune response, and this is the immune system's signal that this is a self antigen. This is also important for vaccine development because in most vaccines, in addition to having some antigen that's a part of the infectious agent, there's also something called an adjuvant, which is basically something that activates the innate immune system. So the adjuvant activates the innate immune response, and that's important because if you just had the vaccine with just the antigen, there wouldn't be nearly as robust a response. So you need both to activate the adaptive immune system, but also the innate immune system to really get a robust response.
So I want to end by talking about this year's Nobel Prize work, and it involves another mechanism that basically prevents autoimmunity and downregulates the activity of these T cells, and that involves another type of-- we've only talked about activating receptors on the T cell, right? The T cell receptor, CD4, CD8-- they're activating receptors for the T cell receptor, but there are also inhibitory receptors that are on the surface of T cells. So inhibitory-- we'll just call them receptors. One is called CTLA4 and another is called PD1.
Their names are not terribly important, but what they do is they keep the immune system in check. And we've talked a lot about signaling and how signaling gets activated, and often a step in signaling is once you get the signal sent, and it's been sent, there is, like, a negative feedback that then turns off the signal such that there's signal termination. So you often have some type of signal termination. That way, you don't have just a constitutive activation of the signal, which in this case would be sort of inflammation and an immune response, and one or both of these is involved in sort of keeping the immune system in check and stopping it after you get that initial reaction.
Now, the reason this is so important and why James Allison and Tasuku Honjo won the Nobel Prize is they had the idea to use this as a therapy for cancer. And it turns out that some cancer cells can express the ligand for these inhibitory receptors such that they can avoid the immune system from recognizing the tumor. So this would be one case where the tumor cell is expressing the ligand for PD1, and that inhibits the function of this T cell receptor so that it doesn't kill the tumor cell, and that leads to the expansion of the tumor so the tumor can expand in an unchecked way.
And what James Allison and Tasuku Honjo determined is that if you block that inhibitory receptor, then you sort of uncheck the response of the immune system such that these immune cells are now able to recognize the tumor cells and kill them. So by sort of blocking the inhibitor, you now have T cells-- these are CD8 positive T cells that are killer cells-- they will now recognize these T cells and kill them off. So there's what's known as an inhibitor blockade because you're blocking the inhibitor, and these inhibitors are antibodies that recognize these inhibitory receptors, and they're now being used to treat some forms of advanced cancer.
And so this is something that the cancer field and immunology fields are both really excited about. What might be one complication with this type of treatment? If you get rid of the inhibitory receptors, what might be a consequence? Yeah, Steven?
AUDIENCE: Then you could recognize other self cells that inhibited [INAUDIBLE].
PROFESSOR: Yeah, you get autoimmunity. That's exactly right. So one of the downsides of this is that-- one of the side effects is that you can have patients with an autoimmune reaction. So it's not the magic bullet, but it's a step in the right direction. All right, we'll see you next week.