Flash and JavaScript are required for this feature.
Download the video from Internet Archive.
Description
Professor Martin introduces the topic of immunity, defined as resistance to disease based on prior exposure. Beginning with vaccines as an example, he gives an overview of the immune system, followed by its properties of specificity, diversity, and memory.
Instructor: Adam Martin
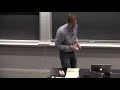
Lecture 30: Immunology 1—...
ADAM MARTIN: And today, we're going to talk about immunity, which is important, especially at this time of the year. So immunity is the resistance to disease based on a prior exposure. Based on prior exposure. And of course, this is the principle behind vaccination.
So humans have been sort of using the properties of the immune system to prevent themselves from getting disease for centuries. One of the first very clear examples of this is back in the 18th century with the English physician, Edward Jenner, and Edward Jenner found out or came to the realization that farm hands on farms, specifically milk maids, that were exposed to a variant of smallpox from cows, which is known as cow pox, could become immune to smallpox.
So cow pox is a less severe form of the disease. And what Jenner did was to take pustules from individuals who had the cow pox disease and inject them into an eight-year-old boy, and then infect that boy with smallpox to show that the boy was immune to smallpox after having received the cow pox material. And so this is the first example of the vaccine. And because the vaccine was derived from basically someone with cow pox, the word vaccine is from the Latin root of vaca, which is cow, so that's where the word vaccine comes from, OK?
So today, we're going to talk about the systems that our bodies have to fight disease and there are several different all levels of the immune system. So I'm going to talk about two of them. So I'll talk about two levels of immunity. The first I want to mention is the one that we're all just like born with, which is known as innate immunity.
[SNEEZING]
[SNEEZING]
Bless you. So innate immunity, as the name implies, is something that we are born with, so this is inborn.
It also doesn't have a delay in when it's activated, right? So if you have an infection, this is sort of the first line of defense, right? There is an immediate response, and that's the innate immune system, so this is immediate. Here, I'll put this down here. It's immediate.
So one example is of an innate immune response. This is not in the body but ex vivo, but here you see a human neutrophil, and neutrophils are part of our innate immune system. And neutrophils hunt and kill bacteria, right? You see that neutrophil chasing after that bacterium, and it's going for it. It's really trying to get it, but that bacteria really wants to get away, but got it! OK, great.
So these neutrophils are part of the innate immune system. It's inborn, it's immediate. And in addition, the response of the innate immune system doesn't really change if you've been exposed to an infectious agent prior to the-- OK, so this does not change. I'm using the Greek delta for change. Does not change with prior exposure. OK, so it's sort of like a constant surveillance mechanism in your body that will go after foreign agents, OK?
Now, this is very different from the next level of immunity, which is known as adaptive immunity. And as the name adaptive immunity implies, this is a type of immunity that does change. It adapts, OK? And this type of immunity is acquired, so it's also known as acquired immunity, but it's acquired with exposure to a foreign agent, OK?
So this involves a change in immunity, this one does not, but the innate immune response is immediate, whereas adaptive immunity takes time. There's a delay, so this is also delayed. It's also highly specific, OK? So it's highly specific to the foreign agents that you are infected with. The innate immune system is less specific. It'll recognize, like, things like bacteria, but it won't be able to necessarily distinguish between different types of bacteria.
So this is more specific than the innate immune system. This is why every year, you have to get a flu shot, because the flu virus is constantly changing. And our immune system is so specific that unless we get a new vaccination, our bodies will not be able to recognize it, OK?
So this is-- so now I'm going to break down adaptive immunity into two branches. One is known as humoral immunity, and humoral immunity is basically protein-mediated, and there are proteins that mediate this are called antibodies. These antibodies are proteins, and it's called humoral because the antibodies can be secreted into the fluids or humors of our body, which is basically the blood, OK? So there is humoral immunity.
The other type of adaptive immunity is cell mediated, and one thing I want to point out that the types of cells that make an antibody are known as B cells. What the B stands for isn't really important, but one thing that's helpful is that these cells mature in the bone marrow, and B stands for bone marrow, OK? So you can always remember where they mature. Now cell-mediated immunity, in contrast, involves a different type of cell called a T cell, and the T of T cell stands for thymus because these cells mature in the thymus.
And I just want to point out where these cells come from. So we talked about adult stem cells earlier, and in this case, these T and B lymphocytes over here are derived from a multipotent hematopoietic stem cell, which generates a whole bunch of different types of cells. Many of them are involved in the innate immune response, but this common lymphoid progenitor over here gives rise to D-- T lymphocytes and B lymphocytes, which are involved in adaptive immunity. OK, so it's not important that you remember where-- what all these cells come from or what they, like, what the tree is, but that these cells arise from a common progenitor cell.
OK, so both of these branches of the adaptive immune system have what are known as antigen receptors. I'll abbreviate antigen, AG. So they have antigen receptors, meaning that they have things on them that recognize specific antigens, and antigens are basically things that result in an immune response. They could be proteins.
Antigens are substances that activate the immune system. That's just immune system, OK? Another abbreviation that I'll use is when I refer to an antibody, I'm going to abbreviate it, AB.
All right, so we have these two branches of the immune system, and they each have the type of antigen receptor, so now I want to go through what these different types of antigen receptors look like, OK? And I'm going to start with the B-cell antigen receptor, also known as the antibody, also known as an immunoglobulin. OK, so another-- these are all synonymous, but you will see them in different contexts. Immunoglobulin is abbreviated IG.
And what the antibody looks like structurally, it looks like this, and I'll just draw it out for you down here. So I'm drawing a lipid bilayer that represents the plasma membrane. The outside of the cell is going to be up, so that's the exoplasm up here. The inside down here is the cytoplasm. And this would be a B cell, then, we're talking about here. I'm going to draw just a segment of the B cell plasma membrane.
And the B-- the antibody can have a transmembrane domain that spans the plasma membrane, and then there are domains-- and what I'm drawing here is a circle, is an IG domain, so this is going to equal an IG domain. It's just a type of protein fold that is modular, OK? So you can see up on my diagram here, right? You see these like here there are these two green segments labeled V and C. Each of those is a single IG domain. OK, it's just a modular fold that is separate from the other part of the protein.
OK, so here we have along-- this is one polypeptide chain that has a transmembrane domain, and it is inserted into the plasma membrane. The N-terminus is here, the C-terminus is down here, and each antibody protein has two of these long peptides. And because they're the longest part of the molecule, they're known as heavy chains, so these are the heavy chains.
And each antibody protein is composed of two identical heavy chains, OK? So these are identical. And then also there's another component, which is present up here, and this is a shorter polypeptide. And because it's shorter and smaller, it's known as the light chain. OK, that's the light chain.
OK, so that's more or less what an antibody looks like. The part of this antigen receptor that recognizes the antigen are the tips right here, so this is where the antigen binds, and it can bind on either this side or this side. This molecule is laterally symmetric. One side is identical to the other, OK?
Now, the T-cell receptor looks different, and the T cell receptor has fewer names. It's just called the T-cell receptor, or the TCR, for short. And the T-cell receptor is structurally very different, so now I'm drawing here a T-cell plasma membrane. Here's the plasma membrane. The exoplasm, again, is up. The cytoplasm is down below this plasma membrane.
And the T-cell receptor has two chains. One is called alpha and the other is beta, and it has fewer immunoglobulin repeats, so that you can see you just have this sort of smaller system here, where you have an alpha and a beta chain. And in this case, this region here recognizes the antigen, OK? So basically the T-cell receptor, or the tip of it, interacts with the antigen.
Now, the B-cell receptor, or the antibody, has different forms, so let's talk about the different forms. And these are shown up on my slide above, right? So you see over here, here is an antibody that has a transmembrane domain and is anchored in the plasma membrane, but there's another form that lacks that transmembrane domain, and instead of being an integral membrane protein, is instead secreted into the blood, OK?
So the forms of the B cell receptor are both a membrane-bound form, which is initially how this antibody is presented, but later on, it can be secreted, and this often changes when there is an infection, OK? So once you have a virus or bacteria in your system, then you get the B cells sort of pumping out the secreted form of the antibody in order to fight the infection, OK? In contrast, for the T-cell receptor. For the T-cell receptor, there's only one form, which is the membrane-bound form, OK? So for T-cell receptors, it's membrane only.
OK, another thing that differs between these antigen centers receptors is the types of antigens that are recognized. So antibodies can recognize all sorts of different molecules, OK? They're very promiscuous, but they-- and a given antibody is not promiscuous. A given antibody will recognize a very specific structure, but the possibility for antibodies is that they can recognize small molecules. They can recognize proteins, they can recognize DNA, they can recognize carbohydrates, you get the idea, right? They really can recognize a whole range of different types of molecules.
In contrast, the T-cell receptor is more restricted in that T-cell receptors will recognize peptides or short sequences of amino acids. So it recognizes peptides, and these peptides are presented to the T cell on a type of molecule presented by the MHC complex. There are two classes, 1 and 2, and we're going to talk about this in detail in Friday's lecture. So I just want to point out the difference in the types of antigens that can be recognized here, and we'll talk about exactly what that means on Friday.
OK, so now we have to talk about the amazing properties of the immune system. The first is how specific it is, its specificity, and I think this is a really amazing property, the ability to really discriminate between very closely related molecules, right? And this is essential for immunity to work well. You want to recognize things that our foreign agents that have like invaded your system. You don't want to be recognizing proteins and structures that are natively present in your body, because if your immune system did that, you'd have an autoimmune disease, so this specificity is really crucial for the function of the immune system.
So now I want to talk how is it that the immune system achieves such high levels of specificity, and the way I want to illustrate this is I want to bring this down quickly. So if we consider the structure of the antibody, these different domains are different in that-- in how variable they are, so some are variable. So this domain here for the heavy chain is the variable domain of the heavy chain, which I'll just abbreviate VH, and then these other immunoglobulin domains are constant, meaning they don't have a lot of variation in sequence. Like the heavy chain, there is a variable domain for the light chain, which I'll abbreviate VL, and then there is a constant domain for the light chain, OK?
And so what I want to do now is consider what the sequence variation is here on this antibody is the same over here. This is the same thing over here. You have a variable domain for the heavy chain and a variable domain for the light chain. So let's consider the amino acid sequence of the antibody molecule specifically at that variable part of the protein.
So let's say we could take individual antibodies and define their sequence from end to C-terminus. That would be from tip towards the end here. So if we take a number of different antibodies and align their amino acid sequence-- so what I am-- I'm not writing out an amino acid sequence, but I'm just illustrating like a particular type of computational experiment you could do.
So these would be aligned amino acid sequences where each of these represents a different antibody, let's say, heavy chain polypeptide that's produced from a different B cell, OK? So each of these is a different antibody from a unique B cell. And then we just consider the residue number and how much each amino acid residue varies along this sequence.
So if we were to align antibody gene stretches like this and look at how much variation there is, you'd get a graph that looks like this, OK? So the y-axis is the amount of variation and the x-axis here is the residue number along this polypeptide sequence. And what you see, probably even without the color here, is that there are these three regions where there's a lot of variation in the sequence of different antibodies, OK?
So here you see the blue segment here has a lot of variation, the yellow segment has a lot of variation, and the reds segment here has possibly the most variation. And what these are known as are hypervariable regions, meaning that they exhibit a lot of variation. Another name for them is that they are complementarity-determining regions. Complementarity. Complementarity-determining. Determining regions, or CDRs, and there are three of them, 1, 2, and 3, OK?
So there are regions in this antibody molecule which are much more variable than others, OK? So what are these regions? Well, this is a sort of crystal structure of the-- of an antibody, and you can see how the antigen is bound at the end. That would be this end of the molecule or this end of the molecule. And here you see a ribbon diagram of the structure of the antibody, and the complementary complementarity determining regions are the regions here that contact the antigen.
And what they are are basically here's an IG fold, this whole thing, and there are these three loops that extend out of the end of this molecule, and you can think of them as three fingers, OK? Then these fingers are able to reach out and sort of grab on to like a foreign particle and/or any particle and stick to it, OK? So these are the variable regions, and they have differences in amino acids-- in amino acid sequence, and even very small differences in the amino acid sequence at this particular part of the antibody can have a huge effect on whether or not they're able to stick to something, right? You can imagine if I lost my thumb, then right now, I'm not able to sort of stick to that anymore, OK?
So small differences in amino acid sequence result in large changes in the affinity of this antibody for an antigen. And antibodies have different sequences, meaning that they're able to bind to specific substances differently. So if an antibody has one set of sequence, it might recognize one structure. If it has another sequence, it might recognize another structure. So just by changing the sequence at these complementarity-determining regions has a huge influence on what these proteins will bind to, OK?
Now, each B cell expresses a unique antibody and just one unique antibody. So each B cell in our body expresses one and only one antibody protein, and that antibody protein has a unique sequence at the CDR region, and this one antibody has unique specificity for an antigen, OK?
So here you can see in my diagram, I have a whole bunch of B cells here. They all express a different antibody, and you can see that the way you could get more of a given antibody is to clonally expand one of these cells, and all of the cells that result from that colonial expansion will express the exact same antibody. And when you have a clonal population of a cell that all has the same antibody, that's known as monoclonal, OK? So each B cell will have a B-cell receptor or an antibody with unique specificity.
So now the question becomes, OK, so I told you how you get specificity, but in order to have a functioning immune system, you need to have lots of different cells that each express a different cell receptor, so there needs to be a way to generate diversity. And the answer to how we generate diversity has an MIT connection. The research wasn't done at MIT, but the person who discovered the mechanism is now at MIT. This research was performed by Susumu Tonegawa, and Professor Tonegawa, for his work on how this diversity is generated, was awarded the Nobel Prize in medicine in 1987. OK, so Professor Tonegawa did this research elsewhere, but now he is a faculty member here at MIT.
All right, so diversity. The problem of diversity, right? We have millions of B cells that have a unique antibody. OK, so one solution to this problem would be we have a million different antibody genes, and each B cell clone sort of expresses one of them OK how many genes do we have? Anyone know, roughly, on the order of magnitude? Do we have a million? What's that?
AUDIENCE: 30,000?
ADAM MARTIN: Exactly. Yeah, so Mr. George has suggested-- Miles, I believe. Yeah, OK, good. Miles suggested 30,000, which is the good upper limit, right? So having a million antibody genes sounds a little bit unfeasible, OK? And so it's basically unfeasible for us to express as many antibody genes or have as many antibody genes as we have antibodies. We just don't have enough real estate in our genome, OK?
But there's another solution to generate the diversity, which is essentially a form of shuffling. So we have a single heavy chain gene for antibodies, and we have two genes for the light chain, but these genes are composed of multiple gene segments. There are multiple gene segments. Specifically, the segments that make up-- that generate this variable domain is composed of multiple gene segments, and these gene-shaped segments are shuffled during the development of the B cell to give rise to different proteins. OK, so these gene segments are shuffled to generate this diversity.
OK, so now I'm showing you on the top here, this is the human immunoglobulin heavy chain locus here. You can see it's pretty big. There are lots of components. I want you to focus on this.
So there is-- you see in orange, there's this variable gene segment, and there are 45 variable gene segments here. There's this diversity, or D segment here, which there are 23 of, and then there are six of these joining or J segments. OK, so these are all distinct parts of the gene. They're all distinct parts of the exon that encodes this variable region of the antibody, OK?
So you have multiple V, D, and J gene segments. And in order to generate a functional antibody, one V has to be brought together with one D, which has to be brought together with one J for that heavy chain, OK? So you have multiple V-D gene segments, and they have to be brought together to form a functional antibody. OK, that's illustrated right here. So here you see this is the light chain. For the light chain, there are only V and J gene segments. V For the heavy chain, there there's V, D, and J.
And so most of the cells in our body and the cells of our germline, at the very earliest stages of development, all have this arrangement, where you have everything still intact. But during lymphocytes development, specifically in lymphocytes, there is a recombination event that brings together V and J segments or V, D, and J segments, OK? So this is mediated by recombination at the heavy and light chain genes for that antibody, OK?
And so this is very different from the recombination we talked about earlier in the semester, where recombination is happening during meiosis and the formation of the gametes, right? In that case, recombination is happening between homologous chromosomes. Here we're not talking about recombination between homologous chromosomes. We're talking about recombination that brings together and deletes segments along a single chromosome to bring these V and J segments together, OK? So this is sort of a intra-chromosomal recombination, which deletes the intervening sequences and brings these gene segments together to form a functional antibody protein.
So this process is known as V(D)J recombination, and this is lymphocyte specific. OK, and that's because during the development of B and T cells, there is an induction of recombinases that mediate this recombination. So in this case, there is recombination, which is mediated by recombination-activating genes 1 and 2, called RAG1 and 2, OK? So there are these are lymphocyte-specific recombinases which mediate this rearrangement, which bring together a unique V, D, and J segments together, OK?
So the diversity comes from the fact that each of these V, D and J segments, each V segment-- you could-- this also applies to D segments and also J segments-- has a unique sequence. So it encodes for a unique amino acid sequence, meaning that if you bring together different combinations of Vs, Ds, and Js, you get a distinct protein, OK? Now even if you had all of the combinations of V, Ds, and Js, you still don't have the diversity that we see in the human body.
So there is another process that further generates diversity, which is the fact that when these segments are getting shuffled, it's imprecise in that nucleotides can be inserted or deleted as these segments are joined, which generates greater amino acid diversity, and this is called-- it's called junctional imprecision. So this recombination is not precise, but it leads to the insertion or deletion of nucleotides of nucleotides. And if there's a multiple of 3 nucleotides either inserted or deleted, then you get a functional antibody.
Why is it that it has to be a multiple of 3? Jeremy?
AUDIENCE: Otherwise, you end up with a frameshift mutation.
ADAM MARTIN: Exactly. Right? This is and the-- this is on the more sort of like on the N-terminus side of the gene, right? So if you inserted one nucleotide between V and J, then the downstream portion of the gene, the downstream part of the open reading frame would be out of frame and wouldn't generate a functional protein. OK, so it has to be a multiple of 3. Yeah, Georgia?
AUDIENCE: How is functional precision lymphocyte-specific? Or is it not?
ADAM MARTIN: It's just the RAG1 and RAG2 are turned on specifically in the lymphocytes as they mature.
AUDIENCE: And that also affects the insertion, deletion?
ADAM MARTIN: Well, if you don't have recombination, you can't get junctional precision, right? So the junctional imprecision-- or junctional imprecision. The junctional imprecision is a consequence of the recombination process itself, right? So if you're not having recombination, you're not having any junctional imprecision because you're not generating a junction.
OK, now there's one more thing that's important here, which is something that happens not as a consequence of this recombination process but as a consequence of activating the T cell response, which is that in addition to these variations, there's also something known as somatic mutation. So there's an elevated mutation rate at the IG locus that further increases the diversity of the amino acid sequence at these variable regions of the antibody, OK? Another way this is referred to is because it can increase the affinity of the antibody for a antigen, it's also known as affinity maturation, so these are synonymous. Maturation. Maturation. OK, so-- and this depends on the T cell, the cell-mediated branch of adapted immunity, so this is T-cell mediated.
So one other aspect of this process that I want to talk about is until this recombination happens, the immunoglobulin gene is not expressed, so it's this recombination that leads to the expression of the-- either the heavy chain or the light chain gene, OK? And that's because the enhancer is sort of downstream in the gene, and by deleting the intervening sequence here, you bring the promoter in range of the enhancer, and now this gene is expressed, OK? But remember you have two copies of each of these genes. You have a parental copy and a maternal copy, and another feature of this system is that there is what is known as allelic exclusion.
So the system is such that a B cell expresses only one antibody, and so if you had both alleles expressing, that wouldn't be the case, OK? So allelic exclusion makes it that if you get a recombination event that leads to a functional antibody for one of your sort of inherited copies of the gene, one of your alleles, it suppresses recombination on the other one, OK? So you will only get one of these genes, one heavy chain and one light chain, expressed per B cell. OK, so only one gene expressed so that each B cell only has one antibody.
OK, I just wanted to point out, finally, that these junctions between V-D and J segments fall right in this CDR-3 region, so they're responsible for the high level of variability at the CDR or hypervariable 3 region. OK, and because of the allelic exclusion, each B cell expresses only one antibody, OK? So all of the antibody proteins expressed by that cell will be exactly the same.
OK, so now the last property of the immune system we need to talk about is memory. And so the immune system needs to be able to recall past infectious agents that it's experienced, and so it needs-- I guess we're kind of personifying here, but it needs some sort of memory, right? It needs the ability to recall this, OK? And this is the principle behind vaccination, right?
The way vaccines work is to put in one of these attenuated or inactivated foreign agents, such that your body is able to remember that later on when you get the real deal, and it's able to fight it off, OK? So the body has to be able to remember. And several ways in which this manifests itself, if we compare a primary infection, the first time you've seen an infectious agent, versus a secondary infection, they have very different responses from the standpoint of the adaptive immune system, OK?
So if we consider the lag before your adaptive immune system really takes off, the primary response takes about five to 10 days, so it's a bit delayed, whereas the secondary response can be one to three days, OK? So it's faster. It's able to react faster when you see an infectious agent the second time. If we also just consider the magnitude of the response by considering how much antibody, the antibody concentration that's like put into your system, then the primary response is smaller and the magnitude of the secondary response is larger.
So you basically-- your body's able to produce more antibody against an infectious agent the second time it sees it. Not only is the antibody amount better the second time, but actually the antibodies themselves are better antibodies, OK? And we can show that by thinking about antibody affinity, which is how tightly the antibody recognizes the antigen, and I'll give you numbers that represent the dissociation constant for an antibody to a given antigen. So the lower that number is, the tighter the binding.
So for the primary infection, the antibody affinity is weaker on the order of 10 to the negative 7th molar in terms of KD, and this secondary infection generates antibodies that are functionally quite better. They bind much tighter. It can be less than 10 to the negative 11th molar, which is sub-nanomolar. Right? That's a really tight interaction between two molecules. So the antibodies, you get more of them, and they're better antibodies, OK?
So what makes this memorable is that when-- what lasts in your body from the first time you see the agent to the next is there's a type of B cell known as a memory B cell, and this memory B cell will express a given antibody, and that antibody will be specific to the substance you saw previously. And because recombination is-- this recombination is irreversible, then that B cell is going to remember that antibody because it's still encoded in the genome.
So the memory results from V(D)J recombination being irreversible and the fact that these memory B cells stay in your body, even if the antigen is not present, so these also stay in the body. OK, so effective vaccines generate these types of cells, these memory B cells. OK, that's important if you want an effective vaccine, that you have these B cells that retain information about the past infection.
All right, so what exactly is it that the antibodies do? So I'll talk about effector functions of antibodies. So antibodies can bind to a foreign substance and interfere with the normal function, right? If you have a bacteria and maybe the antibody binds to some part of the bacteria to interfere with that bacteria getting into the cell, and this type of effect is known as neutralization. If you had an antibody that bound to something like a bacteria, you could also have it recruit phagocytic cells to internalize that bacteria, and so you could also induce phagocytosis.
In addition, antibodies, when bound to a foreign substance, if that foreign substance is a cell, then it could recruit a killing cell to kill that cell, so there's also a killing aspect to this, OK? So what's in this diagram here is a type of cell known as a natural killer cell that is killing its target cell, and so you can kind of think of this cell as the Terminator, OK? So right, if the natural killer cell recognizes this target here, then it's hasta la vista, baby, and that cell is dead, OK?
I just want to point out one thing that I mentioned before, which is that antibodies can be leveraged to generate treatments for certain types of diseases. And we talked about a drug called Herceptin-- or not a drug but a-- it's an antibody, but it's a treatment for HER2 positive breast cancer, so this is used to treat HER2-positive breast cancer. And it's really been a nice success story in the cancer field because what this-- what Herceptin is-- it was derived from a mouse antibody, so this is a mouse monoclonal antibody that recognizes this HER2 growth factor receptor, which is over expressed on 30% of human breast cancers.
And what Herceptin is is that researchers took this mouse antibody and engineered a human antibody to have the mouse sequence at its complementarity-determining regions, such that you have a human antibody that won't be sort of removed by the human immune system but will recognize HER2 and recruit human immune cells to HER2 positive cells, possibly killing those cells or binding to HER2 and somehow neutralizing the activity of HER2 on these cancer cells. So antibodies can be very useful for therapeutics, as well as being useful in our own bodies to mediate immunity.
OK, we'll talk about T cells on Friday. Remember to bring your projects.