Flash and JavaScript are required for this feature.
Download the video from Internet Archive.
Description: This lecture covers Lewis structures, covalent bonds, and resonance.
Instructor: Jeffrey C. Grossman
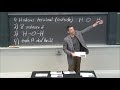
Lecture 9: Lewis Structures I
OK, this is the thing.
We've done a lot of work on ionic bonds.
And I drew the ionic bond energy again, on Monday.
And remember, in an ironic bond-- so let's take sodium chloride-- we have, oh, we're going to go Lewis, right?
And people say, well, why did I draw that dot there.
I could have drawn two dots there and that dot there.
Sure.
Yeah.
But they're kind of looking at each other.
So, you know.
[LAUGHTER]
And remember in an iconic bond chlorine is like, give me!
I want!
And that's the kind of relationship they have, right?
And so this went into something like this, where sodium was like, OK, fine.
Take it.
I'm a plus now.
And chlorine was like, thank you very much.
I'm fully octeted, right?
Like that.
And that's the ionic bond.
So this is ionic.
And we learned this.
OK, but see, there is another kind of bond that can happen.
It's not all about taking and one per-- no.
It can also be about sharing.
About sharing.
So if I have hydrogen instead, let's suppose I have hydrogen like up there.
And I've got hydrogen now.
And here is hydrogen. Well, see when they see-- geshundheit-- each other, a very different thing happens.
Because one of the hydrogens doesn't say, gimme.
And the other one doesn't say, sure.
Well, but something else happens.
Because they get closer.
They get closer, and closer.
Oh!
Let's look at this in terms of like, OK, so here's a plus and minus.
Oh, yeah.
It's not an orbit, it's an orbital!
And here's another plus, and here's another minus.
And these electrons-- you know, the thing is, the electrons are like, I don't like you other electron.
You're repelling me.
And the protons are like, I don't like you other proton.
You're repelling me.
But the electrons do like protons.
And at a certain point, if you bring these together, one of them is like, hey, I got an idea!
What if I'm attracted to you sometimes, over here, and I'm this electron.
I'm like, I want to be attracted to you.
And I'll tell you what, you can be attracted to my proton, and we'll share.
We'll share.
And so, when these come together, what you get is the protons are a certain distance apart, that's set by how far you can push them in without them feeling that repulsion.
And the electrons-- as long as they don't get like, you know super on top of each other, because they're going to repel each other-- they're happier.
They're happier because they got two positive charges that they can kind of go around and be near.
That leads to happiness-- lower energy bond!
But you can see right now, this is a very different kind of bond.
Right?
This is not ionic.
This is covalent.
This is covalent.
When share electrons it's a covalent bond.
OK?
And that is something we write differently.
We write it with a dash.
Well, or you could've also written it-- or maybe you should say, well, let's just put those electrons in between them like that.
Right?
And it's showing that those electrons didn't go to one or the other, they're in between.
They are what are making up the bond together, sharing those protons across the atoms.
OK.
So that's nice.
That's hydrogen. What happens when we get to something a little bit more complicated, right?
So let's go to water.
Now, if I have something like water-- so now, I'm going to go through some examples.
Let's suppose I have water.
Well, I've got this here, and I've got this here.
And now, remember, we're going to think about things in terms of Lewis dots, which I introduced to you on Monday.
Those are the valence electrons in an atom, which are what do all of the chemical bonding.
So those are the ones we're going to carry.
Now, how many dots do I have for oxygen. I forget.
Six!
Six.
And if you didn't know, you know where to look.
Because you're always carrying your periodic table with you-- always!
And so I might write six like that.
OK.
What lines do I draw where?
How are these shared?
So how are the electrons shared in covalent bonds?
Covalent bonds.
And that is where Lewis will help.
That is where Lewis will help.
How do I take these valence configurations of electrons and make a molecule with these kinds of sharing bonds in it?
And there's actually a fairly simple recipe that I want to teach you today, because that's how we're going to do it.
And the recipe is written here.
And don't worry, there's a lot of text here, but I'm going to go through it for three different examples.
And if you haven't done Lewis-- I know some of you have in high school, some of you have not.
That's OK.
If you haven't, practice is really key.
All right?
We'll do three molecules, maybe four, today.
And I encourage you to do a bunch more as practice.
And this is the recipe for drawing Lewis structures.
And Lewis structures is what tells us how electrons are bonded in these molecules.
And so, let's follow this for the one I have up here.
Let's follow this for water.
OK?
So we're going to go through this recipe.
Now, the first-- so, one through six.
I will write one through six for the example.
I will parallel that recipe.
So first, connect atom central.
Central atom is often the least electronegative-- already I'm stuck!
Because I didn't tell you anything about electronegativity.
That's coming a little later.
Today, I will tell you what electronegativity is.
For now, trust me.
So the first step is connect atoms.
Central atom is often the least electronegative.
OK.
Well, so let's see.
So for water, so step one, the H atoms are going to be terminal.
That means that they're on the outside.
Terminal.
OK?
Outside.
They're also called terminal.
And so, the arrangement should be something like H, O, and H.
OK, good.
So that's going to be my arrangement.
OK.
Step two-- determine the total number of valence electrons.
OK, I can do that.
So two.
Well, I've got one, two , three-- OK.
I've got eight valence electrons.
Good.
OK.
Step three is where it gets really fun.
Place bonding pair of electrons.
Go all in, just like I did for-- So there's a bonding pair right there.
There they are.
Two electrons in a bonding pair.
So place bonding pair between adjacent atoms.
OK.
I can do that.
So let's see.
Here we go.
H, O, H. Right?
OK.
So there's my bonding pair.
I put two electrons.
But now, you see, OK, I only used up four electrons there.
OK?
Now, starting with that, now add enough electrons to each one to form an octet.
Start with terminal atoms, add enough electrons to form an octet.
OK?
So let's see.
But hydrogen is special, because hydrogen's octet is just two.
Hydrogen doesn't want eight.
Hydrogen just wants two.
So we called it out here, in the recipe.
Two for hydrogen. OK.
So add enough electrons to form an octet.
Well see, each H atom already has-- Remember they're sharing.
They're sharing.
OK?
So each H atom already has the two electrons that it wants.
And so, for four I've got that-- oh, I started with the terminal.
Atoms.
OK.
And so for four, I've got each H is OK.
It has two.
All right?
It has two electrons, which is what it wants in its valence.
Next one.
If-- oh!
Now, it gets-- If there's electrons left over put them on the central atom.
OK.
So I went from the terminal in.
I went from the outside in.
H has two.
H has two.
That's good.
Oh!
But how do I know if I have any left over?
Well, I did that in step two.
I had a total number of electrons.
Now, how many have I used?
I've used two here, and I've used two here.
I have four left over, because I started with eight.
Right?
And I was just told from step five to put those on the central atom.
OK.
So step five now, is going to make this look interesting.
So H, O, H-- and now, I'm not going to put them in a bond.
Right?
I'm just going to put them on the atom.
And those are called non-bonding.
Well, OK.
That's a genius name.
Nonbinding.
Because they're not bonding.
[LAUGHS]
That's a good name for those electrons.
You can also call those lone pairs, because they're lone.
They're not involved in a bond.
All right?
So those are my four extra electrons.
And then finally, if the central atom is less than an octet, used the lone pairs from terminal atoms-- Oh, this looks complicated.
I don't want to deal with it.
Why?
Because I've got an octet here already.
So six is not needed.
Because everything since the oxygen-- since O is happy.
It's got its octet.
So that is a very slow-- we went slow.
We'll go faster now.
Right?
So that is a very slow introduction to how to do a Lewis dot diagram for a molecule.
In this case, H2O.
Now, you may notice I drew it linear.
Some of us know that is not the shape of this molecule.
But shapes come later.
Shapes come next week, right?
Right now we're not thinking about shapes.
Let's do another example.
So my next example is O, Cl.
I'm taking this and I'm putting a charge on it.
So here's my next example.
Well, anyway, that's my example.
I'm going to go through the numbers again.
We're going to go a little faster.
So I want to know how this looks in a Lewis.
Structure well, OK.
So there's only two atoms, so-- Maybe I should have called this example B, because now I have point 1, all right?
So there's only two atoms.
So there's no central atom.
OK, that sounds like that might be easy.
And then step two is, how many valence electrons are there?
Well, there's six, plus seven, plus one, right?
So oxygen is six.
Chlorine is seven, plus one, equals 14 electrons.
Where did the one come from?
That's because it's got a negative charge here.
It's a negatively charged molecule.
OK.
Good.
Now-- OK, now it gets interesting.
Place bonding pair of electrons between adjacent atoms.
So my adjacent atoms are these two atoms, which I could write like this, or I could also have written it like this.
OK?
Those are equivalent.
Now step four.
OK.
Now, starting with the terminal atoms, add enough electrons to each one to form an octet.
So let's go through this.
So now step four.
OK.
Now, this can count the two electrons.
This can count those two electrons.
You see how this works?
Because we're sharing now.
No one took.
We're sharing.
So they can each count those, because of what I drew up there in terms of how a convenient bond works.
But now, they need another six to form an octet.
So how many?
I used two.
How many do I have left?
I've got 12 left.
It's perfect.
Right?
It's perfect.
So I've got exactly as many as I want.
And I can write O has all of those.
Cl has all of these.
And if we want to be careful about it, we will emphasize that this molecule is not neutral.
It got an extra electron.
That's why we put the minus sign up there.
Right?
It's charged.
And this molecule, OK.
Each atom-- let's see, OK.
I used the extra electrons.
If electrons are left over-- No electrons left over.
If central atoms-- no, they're already octets.
So five and six are not needed.
Oh, but you know the last example is going to be more interesting.
So let's do that.
Last example.
O-- C, example C. O-- so I've got H, OK.
I'm just going to write the atoms here, because I'm starting where I don't even know what it's going to look like.
I don't even want to hint at it.
Those are my atoms.
I've got two hydrogens, an oxygen, and a chlorine.
OK.
I'm sorry, a carbon.
Oh, yeah.
I meant this to be a carbon.
There we go.
Two hydrogens, an oxygen, and carbon.
OK.
Now, as we will see when I tell you what electronegativity is, carbon has the lowest electronegativity.
OK?
And so, I'm going to put that in the center.
That's going to tell me that if I answer point 1, it might look something like this.
Oh, now it's taking some form, right?
OK?
So the hydrogens are going to go like that.
Their terminally oxygen is outside, carbons in the middle.
And how many electrons do I have?
OK, so now, let's see.
Answering step two, I've got 12 valence electrons.
OK.
Because I've got six, four, five, right?
So 12.
OK?
Good.
And now, I'm going to put the bonding pair in there.
So let's do that.
So step three-- OK, so now I've got C, H, H, O. So I've done step three.
OK.
So I've got two, four, six.
I've used up six electrons in this sharing covalent bond situation, and I started with 12.
So that means I've got six left.
OK.
So I'm going to go to step four.
Now, starting with the terminal atoms, add enough to each one to form an octet.
Now hydrogen is good, right?
Because hydrogen, remember, it's octet is a doublet.
It's two.
So that's good.
But what about oxygen?
OK.
It looks like oxygen needs some.
So now, we're going to write this structure like this.
I've got to add six more electrons to oxygen in order to fulfill its octet, right?
And oxygen is happy.
All right?
Yeah, it is.
Just like that.
But if electrons are left over-- but are there any electrons left over?
No.
So I can't place electrons on the center atom, yet now we invoke number six.
If central less than an octet-- it is two, two, two-- then use lone pairs from terminal atoms to form multiple bonds to the central atom.
Oh, yeah.
Now we're there.
Now we're there.
So now we jump to step six.
Because look.
Let's continue.
Because look, if I add all these up and I go to carbon, it's not happy.
But let's make it happy, because that's what Lewis lets us do.
And I'm going to go down to here-- six continued.
And all I need to do to do that is to follow the instructions, which is, taking one lone pair and put it here.
So I'm going to draw these explicitly now as dots, right?
So I took one of those lone pairs and I moved it here.
And I created a stronger sharing bond.
I wrote down the guidelines of Lewis, remember, on Monday.
And the third point was octet!
Right?
Atoms want to achieve their nearest octet.
And this this tells us then, by following these steps to implement Lewis' dream and vision, we get nothing less than the nature of bonding itself in molecules.
That's cool.
And so you could write this with sticks if you want.
C, H-- that's a double bond with those electrons up there.
It's the same thing.
And now, if you count-- happy, happy, happy, happy.
Everybody's happy.
Caring is sharing.
They're all sharing.
So this is the general idea of Lewis.
Following the Lewis prescription tells us about covalent bonds, and it tells us how many electrons are going to be shared.
Now, it also tells us something else that's very important, and it's through this concept called formal charge.
So that's the next thing that we need to learn.
Because now, I go to another molecule.
In this case, it's formaldehyde.
OK.
CH2O.
But look, now, neither of these is done.
These are not done.
But it's like my starting place.
OK.
Carbon central here.
It's kind of central-ish there.
Can I start with either of these?
And the answer is yes, you can.
And you can draw-- and let's do that-- you can actually draw correct Lewis structures.
Let me use the board over here.
All right?
So I could draw this like this.
H-- Let me see here.
H, C, double bond, O, hydrogen. And I could put that there and that there.
Or I could draw it like this.
Do I have it this way?
Yes.
All right?
Like this.
And carbon double bond O.
And when I look at this, and I look at the electron count, it all works out.
All right?
I got to add my lone pairs to the oxygen.
Don't let me forget those.
It all works out.
The total number of valence electrons is 12 in both cases, and everybody's obeying the octet rule.
But the concept of formal charge allows us to distinguish between which one is likely to be more stable.
And so, what formal charge is-- and I've got it written out here-- OK?
So formal charge-- non-binding electrons count for the given atom.
Bonding electrons are divided equally.
And so formal charge is the total valence of an atom-- so I can take atom here and say, what's it's total valence?
Carbon, four.
Oxygen, six.
All right?
So that's the total balance of the free atom.
And then I subtract from that how many nonbinding electrons it has, plus the bonding ones over two.
Right?
And so, if I do that-- let's write that.
So if I think about that-- gesundheit-- from my pictures, the formal charge is equal to the number of valence electrons of the free atom.
That means the atom, before it ever participated in a bond, minus the number of dots, minus the number of lines.
Because that is really the same definition, right?
That's the same definition.
So let's go up to this one.
So now, if I look at carbon in this configuration, and I calculate the formal charge of carbon, it's going to be 4-- the valence electron of the free atom-- minus 2-- because it's got the two dots there, right?
Minus 3-- 1, 2, 3.
Or the total number of electrons involved in bonds divided by two-- 6 over 2.
3, right?
So those are equivalent.
But that means that the formal charge on this is-- just spell it out here for now-- formal charge is equal to -1.
And if I do the same thing here, then it's +1.
But you see, if I look at this structure, and I think about the formal charge on the carbon, atom-- Again, I'm basically counting a change from the free atom.
OK?
And so carbon had four.
What did this new bonding environment do to the valence chemistry?
And in this case, the formal charge on carbon is going to be 4-- starting with the number of free valence electrons-- minus no dots, but it's got one, two, three, four bonds.
So that's zero.
And the formal charge on the oxygen is going to be 6 minus 1, 2, 3, 4, 5, 6.
So that's also zero.
And you get a sense-- how much did I push this atom away from where it normally thinks about its charge balance?
And you get a sense for why this might be true.
Lewis structure with a set of formal charges closest to 0 is usually the most stable.
And that's our guideline.
And so, this one not very happy.
That one happy.
And so you can calculate the formal charge on any atom in any Lewis structure-- gesundheit-- by following this very simple procedure.
You take any atom and you look at how you've drawn it's Lewis structure, right?
And you just count.
So for example, if I take CO2, and I think, well, I can do the same thing here.
I've got two Lewis structures and they look like they both satisfy the recipe.
The recipe is satisfied.
But which one is more stable?
Well, if I look at this, it's the same idea.
And I won't go through it in detail, but if I look at this then-- C, O, O, with lone pairs on the Os, like that.
All right?
This is going to give me formal charges of 0, 0, and 0.
Whereas, if I do the one on the right and I put a triple bond-- so I put too many electrons sharing-- then yeah, I satisfied the octets, but no, I'm not as stable, because the formal charges aren't as close to zero.
So this gives me to my brief, why this matters for take.
Why would I care which one of these CO2 takes?
All right?
OK.
Well, it turns out that as long as we're not at absolute zero, this molecule is moving.
And so, obviously, that's related to this.
It's not obvious.
But cars in the US are now the number one emitter of CO2.
OK?
They're the number one cause of CO2 emissions.
And here's a nice little corner somewhere.
And so, this is coming out now from the transportation sector.
More of the CO2 comes from that than any other sector.
Why does it matter?
Well, why does this Lewis structure matter?
Well, it has to do with how it moves.
Because, you see, I showed you this before.
And I didn't complete it because we were talking about electron transitions, and then I gave you the example of ozone being really important for absorbing in the UV.
And I showed you the chemistry of ozone degradation with CFCs.
You see, look at this absorption out here.
There's CO2, right?
And so, there's the sunlight above the atmosphere.
Here it is on Earth.
And you could see CO2 there.
But see, the reason CO2 absorbs there has to do with how it moves.
It has to do with its vibrations.
Now, that's not something that you need to know for like a test or something, but I wanted to tell you about it because it is directly related to what we just did.
Those vibrations-- and by the way, that first mode is the one out here doing all the IR absorption.
Right?
This one.
And you can see right away, if this thing is wiggling, it's going to wiggle very differently.
Right?
It's going to wiggle very differently whether it has two double bonds on either side of the carbon, or a triple and a single.
Right?
And so that, alone, tells you something really important about how it interacts in our atmosphere with radiant heat, right?
With IR radiation.
OK.
That's my 'why this matters.' Now, I've been talking about all these things in absolutes.
But you see, the world is not so absolute.
And in fact, we're missing a really important part of the picture here.
So this is H2, right?
There is an iconic bond where, remember, it's like, I'm picky!
OK.
And then it's like a Coulomb interaction.
And here it's a sharing.
Each electron sees both of those protons.
But there's a whole bunch of room in between, right?
There's a whole bunch of room in between.
And so what we need next, as we talk about covalent bonds, is we need a way to think about how ionic they are.
Right?
Because there's only a certain kind of covalent bond that's purely covalent.
As it's written here-- non-polar covalent.
So let's talk about that next.
And what we're going to do is use this term that I alluded to in the beginning, which is point number one in your recipe, and that is electronegativity.
OK?
So I'm going to get it done.
Almost.
Practice makes perfect.
OK.
All right.
So there is a symbol kai, that we use for this concept of electronegativity.
Electronegativity.
OK?
Now, this is the tendency of an atom to attract shared pair of electrons to itself.
OK?
Let's write "in a bond," just to be sure.
All right?
In a bond.
So if an electron in a bond, the question is, how much was it able to pull those bonding electrons to it?
All right?
And you can already think about this in terms of concepts that we've learned already.
Right?
Like the size of an atom.
How far out electrons are?
Right?
The radius of an atom.
Whether there's shielding going on.
Right?
This kind of leads you-- how many protons there are.
All right?
This leads you already to be able to think about this.
Which atom is going to want a pair of electrons in a bond, right?
Which one is going to want it more, and how much?
But it was Pauling who said, no, I want to go further that.
I want to write down-- even if it's empirical-- I want to write down some way, some number.
I want to quantify this.
And so he came up with a scale for electronegativity.
The term and concept of electronegativity goes back long before Pauling, to, I think, [INAUDIBLE]..
But Pauling is the one who said, I want to quantify this.
And the way we're going to do is we're going to measure bonding energies between all sorts of different atoms.
And what he found is that, if a bonds to a, and b bonds to b, a to b is not just a simple sum of the two.
And that clued him in for how to think about this partially covalent or polar covalent nature.
I'll tell you why that word "polar" is there in a few minutes.
And so Pauling developed this scale-- the electronegativity scale-- to tell us this.
And he arbitrarily set fluorine to four and hydrogen at first 2.1, now it's 2.2.
But basically, this is just some of the elements of the periodic table, and these are their electronegativities.
OK?
And you don't need to worry about how this scale was developed quantitatively.
There are actually many electronegativity scales.
OK?
What I want you to know is what it means conceptually, and then how to use it to think about whether a bond is going to be ionic, or covalent, or somewhere in between.
And so, you can see already here, OK, the extremes of this are fluorine and caesium.
These are the most electronegative, least electronegative, or if you want to be positive about it, most electropositive.
When you're talking to these elements you want them to feel good.
They're not the least.
They're very important.
So they're electropositive.
Right?
And now you know conceptually.
But look, now we also can think about differences.
Because, if the difference between this concept, from one atom to another is zero, well then, that must be a pure covalent bond.
Because neither one could bring in the electron pair more than the other, right?
So like chlorine two-- the chlorine dimer-- then the change in electronegativity between one chlorine and another is zero, it's a pure covalent.
I mean, just think about that conceptually, right?
So that's what electronegativity means.
So if I have two that are the same, neither one can draw in.
They have the same kai.
Neither one can draw the pair towards them more.
Right?
So the same is true for any dimer like this, where it's the same atom.
The H2 dimer that we started with, also has delta kai of 0.
Those are called pure covalent, because it's just pure sharing.
Nobody took more charge than the other.
But see, if I go to something like sodium chloride, well now, from this table I can calculate the difference in electronegativity between sodium and chlorine.
And it's 2.23, which is pretty high.
And it's ionic.
So if that difference is high, then it means one of the atoms grabbed the electrons.
And if it's kind of 0, it's pure covalent.
And if it's somewhere in between, it's this polar covalent.
So, for example, HCL.
So these are all with the same atom-- chlorine.
But here delta kai is equal to 0.96.
So this is called polar covalent.
OK?
So this is also partially ionic, right?
These mean the same things.
There's ionic character-- is another way that we say it.
This bond is not purely covalent because one of them took a little more charge, because it's electronegativity was higher.
And therefore, there's a little bit of that kind of ionic thing going on, because you got a little plus over here, and a little minus over there.
Right?
And that is something that can be looked at for a whole bunch of different bonds.
And so here, this is what Pauling was trying to fit.
So he came up with these empirical fits.
Right?
OK, things kind of tend to lie on this line, which is the plot of the electronegativity difference between two different atoms the percent ionic character.
Is it really fully ionic?
Is it is it fully covalent?
And you can see, over here, it's kind of not interesting, right?
It's just anything with itself is zero.
And over here, you've got some of the same ionic bonds that we've already talked about, right?
And then you've got all this stuff in between.
And what this says is, as you've now seen, right?
[INAUDIBLE] is a suggestion of a rule, and then it gets broken 20% of the time.
This is also a way of qualitatively classifying bonds and thinking about the ionic character in a bond, but it is not always quantitative.
But in general, what you'll see is that if delta kai is greater than 2, then it tends to be an ionic bond.
And if delta kai is less than, let's say, 1.6, it tends to be covalent or polar covalent.
And in-between it depends.
How do I know?
Right?
I'll show you an example.
Where's my example?
I've got an example here.
Sodium bromide.
It's not on there.
But if I take sodium bromide and I take HF, they both have delta kai equal 1.9.
Now, some textbooks will simply say 1.7.
You will see that in some textbooks, because they just want it to be all or nothing.
It's always ionic, or it's-- But the fact of the matter is that in this intermediate regime you get variations that depend on other things.
And so, for example, HF is a gas at room temperature.
So these have the same electronegativity difference, but this really behaves like a polar covalent molecule.
And this really behaves like an ionic solid-- an ionic bond.
How do you know?
It's the properties.
Right?
Remember, I showed you the properties of ionic solids on Monday.
And so, I can look at those and check them off-- solid at room temperature, et cetera.
And I can see, well, does this fit the bill?
Does it look like an iconic solid?
It absolutely does not.
So even though this is the same, you got to be careful in this intermediate regime.
Now, you can also go even further, and you can get quantitative.
Because, you see, what we're really talking about here is something called a dipole.
That is why it's called polar covalent.
OK?
It's called polar covalent because we made a dipole.
And as far as I know, from my electricity and magnetism training, the dipole moment-- mu, it's written as mu-- is the charge times the distance.
Where Q is that the charge.
In this case, it's literally the charge that was pulled.
It's exactly that.
There's the chart, right?
Tendency to pull a shared electron.
I pulled it over here, so I created a little more negative charge on me, and a little positive charge on there.
And that meant that I've got a partial charge now, separated by a distance.
Right?
And so you can actually use this to get quantitative.
So let's do sodium chloride, right?
So for sodium chloride the dipole moment is nine to debye.
And this is just one debye.
This is charge times distance, so one debye is equal to 3.3.
10 to the minus 30th coulomb meters.
That's the units of a dipole moment-- charge times distance.
So if I take sodium chloride and I tell you that its dipole moment is nine debye, then I also have to tell you that the distance between them, r, is equal to 2.36 angstroms.
That is this distance here.
I've made a bond between these two things, and that bond is some distance apart.
Well, that's exactly the distance I need to think about the dipole, right?
So remember, a dipole is because I've got positive charge and negative charge.
And in fact, you might see it written like this.
You might see there's a little positive charge here, and a little negative charge there, and the dipole moment goes like that.
All right?
There's a dipole.
And now, those charges at this distance in the bond allow me to actually be quantitative, because Q is equal to mu over r.
And if I plug that in, then it's 1.3 times 10 to the minus 19th coulombs.
So for sodium chloride-- because I was given the dipole moment in that molecule, and I can look up the bonds distance-- I can actually tell you how much charge are on those atoms if I know that information.
But I also know what the charge of a full electron is.
This is almost there.
It's something like 80% of an electron.
And so that's a pretty strong ionic bond.
But it's not 100%, right?
So this is the message I want to tell you.
In reality, we've been talking about absolutes.
I fully took a charge.
Right?
No!
You didn't.
You took 80%.
And there's a little bit still over there.
Right?
But we do like to still categorize materials.
And that's what this does really nicely, is it says, well, this is going to behave like an iconic solid.
Meaning, it's going to have those properties that I showed you on Monday.
Right?
Even though it's not fully ionic, it's pretty darn ionic.
Right?
And now, even though these are not fully covalent, meaning, pure, non-polar covalent-- non-polar, no dipole in the bond.
But these have some dipole, but that's OK.
They're still acting like covalent molecules.
Right?
And so there's this spectrum in between.
And you can use these concepts to think about the bonding, and to think about in particular this-- that so many bonds are actually like this.
Even though we categorize them like this, and we think about them like that, they actually look like this.
And electronegativity and dipoles are what help us characterize these differences.
Now, there is more to Lewis that I will talk about on Friday.
But there's something called resonant structures, and that's a very important part of Lewis.
I'm going to talk about it Friday, and it will not be on the exam on Monday.
OK?
But on Friday, we'll talk about resonance structures, we'll do a few more Lewis examples, and then I'll talk about exam one.