Flash and JavaScript are required for this feature.
Download the video from Internet Archive.
Description: The VSEPR model allows us to predict the shapes of molecules to more fully understand their properties.
Instructor: Jeffrey C. Grossman
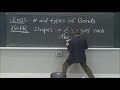
Lecture 11: Shapes of Molec...
Let's get started.
We'll cut right to the chase.
How did it go on the exam?
I crushed that.
I heard I crushed that.
Yeah.
Look at that.
So you guys rocked it on exam one, and I'm very proud of you, and I think this is awesome.
The average was actually really high, which is great.
It means, to me, that you guys have learned the materials that we want you to learn in the first third of this class.
You know, this is also a chance that if you didn't get up into this range, you're here or down here, or even if you're up here, and there are a couple of problems you didn't quite get, this is the time to go back and say, what did I miss?
Right.
How did I misinterpret?
Or what did I miss, and go back over it now.
Solidify this knowledge.
Why?
Because of what's coming next.
And I really want to contextualize this with something really important to me.
And that's bread.
Now, if you want to get the best ride in town you go to Bricco.
All right, and I really actually strongly encourage you all to go to Bricco, because it's true.
It's really the best bread in town.
There's Frank.
He founded Bricco.
It's in the South end.
It's amazing.
But you notice his ingredients, there's only seven ingredients in the bread at Bricco.
There's salt, right, yeast, water.
There.
Anybody know what farina is?
Flour.
OK, crusca, it's like kind of wheat stuff.
Oil and passione.
Passione.
Passion.
It's the thing he's holding.
That's ingredient number seven at Bricco, which is the best friend in town.
Now, here's my point.
I need you guys to keep bringing the passion to this class.
You should be bringing the passion to everything you do, because that's what we do at MIT, right?
That's how we approach everything we do.
But I need you not to say, well, I did really well in exam one, and now I can kind of phone it in.
That's not the time to do it.
Trust me.
Because now we're going into the next phase, the next third, a little bit less than a third of the class where we're going to come maybe out of some of our comfort zones.
All right.
So some of you have seen a lot of the material that we've already covered.
Some of you haven't.
And some of you may have seen some of the material we're about to cover in the next week or two, shapes of molecules and molecular orbital theory, hybridization.
But then we're going to go into crystals, and we're going to take molecular orbitals, and we're going to make solid orbitals out of them, which are called bands, which is going to give us semiconductors.
And then we're going to dope those with chemistry.
All sorts of stuff.
So keep up the passion, please.
That's why I was showing you Frank and Bricco.
Keep up the passion.
OK, now today, we're going to cover a really important follow up to Lewis, which is, how to predict what shape a molecule will be.
And so, you know, if I take, so for example, H2Be and H20, now those look-- right, OK, so, H Be H and H O H, right, from Lewis, we don't really know why they both looked that way.
Are they both linear?
Are the ones below both linear?
How do we tell what shape they are?
And as I'll tell you about today, so I'm going to give you a way to do that and then a recipe, another recipe, just like Lewis, and then, we're going to talk about the goody bag, which allows you to touch and feel the shapes.
You can't-- we're going beyond 2D.
We're coming out of the board.
We're going 3D.
You got to hold it in your hand.
Now, unfortunately, we couldn't buy like 500 kits that are this big.
But I'll pass these around, because you can just feel-- in these molecules, you can just feel that if I do this, it's a different molecule.
If I rotate that, it's a different molecule.
It's actually going to have different properties.
And so that's what we learned today.
Lewis, this is just all flattened into the board.
Doesn't tell me enough.
It doesn't tell-- I need to know what those-- is it like that?
I don't know.
Do the yellow things want to be that close, or should it maybe be like that or like that?
You see how when you can do this?
I could do this for hours honestly.
So I'll pass these around.
And I'm certain at some point we're going to hear loud clinking sounds of these balls falling apart.
They're not held together too well.
Please try to not-- just play with it a little bit.
Right, and then your kit is a smaller version of that.
OK, so how do we do this?
How do I tell you what shape that should be?
Well, we have a way to do this, and it's got a name even.
So just to contrast, Lewis is what we did last.
And Lewis gives me the numbers and type of bonds, OK.
The number and type of bonds.
But now, I've got a way that's called Valence-Shell Electron-Pair Repulsion model, VSEPR, VSEPR.
But see, chemists are-- if I haven't conveyed this before, chemists are really good at naming things.
So it could be valence electron shell pair repulsion model.
It's not.
But it could be.
And if it weren't we'd call it VESPR.
It would sound a lot better.
Right, and so that's what we call it even though we write VSEPR.
So we write it like this, but we say vesper, because this is chemistry.
And this gives us the shape.
Now, it's based on actually a very straightforward premise, which is that electrons repel each other, something we already knew.
OK, electrons repel each other, and the stable arrangement-- I hear that molecule changing shape.
It makes me happy.
OK, the stable arrangement minimizes-- now, this makes sense right?
Minimizes the repulsions.
OK, that's really the whole premise of VSEPR.
VSEPR theory is a way-- it's a very simple recipe that we will learn and apply that is based on this premise.
We already talked about electrons repelling each other in atoms, right, and in a bond.
And now we use the same idea that electrons repel each other for a molecule, right, within a molecule.
OK, good.
But we need to rank order this.
We need to rank order this.
And so we have a rank order.
And you'll see kind of why, as we go.
I'll tell it to you right now.
And I need abbreviations, because I don't want to keep writing bonding pair and lone pair.
So I'm going to say that a bonding pair, a bonding pair, oh what's a bonding pair?
Well, it's the two electrons in a bond right there.
That's a bonding pair.
OK, good.
So a bonding pair, to save time, we're going to write as a BP.
And a lone pair, what's a long pair?
Well, we already know that.
A lone pair is right there.
A non-bonding pair, a lone pair, an LP.
OK, good.
Now, this allows me, now that I have this very important key, I can tell you what the repulsion order is without writing out bonding and lone all the time.
Repulsion order and this is what we follow in VSEPR.
OK, so we're going to go the lowest repulsion is between two bonding pairs, bonding pair to bonding pair.
And the medium medium, OK, so the next in our list is bonding pair to lone pair, and the highest is between two lone pairs.
All I'm telling you is in this ordering is that OK, electrons repel each other, yeah, got it, but there's an ordering to it.
If I'm a lone pair, and I see another lone pair, that I'm more repelled.
Those two things are more repelled, then a lone pair and a bonding pair, which are more repelled than two bonding pairs, and we got one more thing we got to think about, because you see the bonding pairs can be single, double, or triple.
And so those have a rank order, which also makes a lot of sense, right.
So like one bonding pair would have a single bond.
With a single bond would have a lower repulsion than a double bond, which would have less repulsion than a triple bond, right?
But that makes a lot of sense, right?
You're putting more electrons in the bond.
And so there's more stuff to repel.
Right, so if I'm talking about bonding pairs, there is also a sub order.
OK, that makes sense, right?
So this is like a single bond line, two lines, no room, three lines, right?
All right.
Now this frames the VSEPR model.
Now let's apply it, and the rules are actually really pretty straightforward.
So what we're going to do is we're going to build our understanding by applying this to some examples, because it's exactly the same thing we did with Lewis.
OK, so I'm going to start my recipe with the first three ingredients.
Passion is always there, too.
But these are the first three ingredients of my VSEPR recipe.
First, I write the structure.
We know how to do that.
We learn how to do that.
And then I'm going to classify the pairs of electrons as bonding or non-bonding.
So I'm going to just label them like that.
And then I'm going to maximize the separation between domains and pay attention to these rules, and I'm going to start a little bit of a table over here, number-- because we're going to fill this table out-- number of electron regions.
OK, let's do this.
You know when I do this, there's nothing but fun coming.
Nothing but fun.
I went all the way, all the way over.
Why?
Because I need some space.
Now, here, we're going to say electron pair geometry.
We'll be talking about this today, geometry.
OK, good.
And then, here, OK, now this is sort of the molecular-- molecular geometry.
So I'm going from-- I'm going from counting electron regions and labeling what kind they are to a geometry of the electron cloud around things to an actual molecular shape, which is what VSEPR gives us, and to know why is this so wide, well, because there's options in here.
Right, because I could have no lone pairs.
All right.
I could have one lone pair.
And this will all make a lot of sense.
Two lone pairs and so on.
We'll keep going as we get there.
OK, so I'm going to start with a very simple case.
I'm going to start with this.
Right.
And then we're going to put some stuff into that table as we go.
All right.
So look if I have H, Be H, then that's already 0.1.
I'm going to write, just like I did with Lewis, I'm going to write the number of the recipe next to what I'm doing on the board.
OK, so one, write Lewis structure.
I did it.
OK, good.
Now two, I've got two electron pairs.
OK, and they're both bonding.
Both are BP.
OK, and so I've got two BP domains.
And as you can see, I've got no LP domains.
And so three, this is going to be max separation, max sep will have to be linear.
There's just no other way about it.
I've only got two electron domains.
I've got no lone pairs.
So two bonding domains, two electron domains.
It's got to be linear.
That's the only way I can go.
So if the number of electron regions is two, which it is for BeH2, OK, and and then the number of-- the electron pair geometry is linear, right, because that maximizes the separations.
And there is no lone pairs, and so it's linear.
You say, well, that really seems redundant.
It is in this case.
It's not going to stay that way, not going to stay that way.
OK.
Good.
One lone pair, no.
No lone pairs.
I mean, if I had two electron regions total, OK, and one of them was a lone pair, it's kind of boring.
I mean, you know, if this were a lone pair here, instead of a bond, well, clearly it's linear.
There's only two atoms.
That's kind of the simplest case.
We won't really talk about that.
Good.
OK, now it's the same kind of thing.
Let me just make sure that there's no confusion here.
And let's not leave this up.
But if I had-- let's say I go back to my first slide there.
OK, so what if I had this one.
It looks more complicated.
Right, it looks more complicated.
So now, OK, so OK, step one O and here you go, here you go.
And there's a point I want to make here, because if I count up the bonding pair domains and the lone pair domains on this one, all right, say well, OK, I've got the bonding pairs here.
I've got some BPs.
I've got two BP domains right?
Here and here, just like I did in H Be H.
But oh, I've got all these lone pairs now.
Don't I have four lone pairs?
No.
And the reason is we have to pick an atom.
In VSEPR, we have to pick an atom.
We're doing this around an atom.
VSEPR applies around an atom.
You pick a central, and I picked carbon as my central atom.
And then you apply VSEPR.
So there's no lone pairs around the carbon.
That doesn't matter for the shape around carbon.
OK, that's really important.
So I pick my central atom.
So now I know there's no lone pairs, no LP, because central atom.
You know what I mean, central atom.
And then finally, three, we're going to get linear because, again, there's only two electron domains.
There's going to electron domains, and the only geometry really that you can take in this case is linear.
No lone pairs, two electron domains.
Good.
And now you know it's about to get fun, because now we're going to go to something more.
Let's go back to our recipe.
There it is.
OK, so I'm going to make room here and do my next one.
I'm going to go a little bit more complicated.
So now I've got BF3.
So B, and we're going to go F, F, F.
And we know we got all these lone pairs out on the F's.
Remember this is one that's electron deficient.
We talked about this last week, electron deficient.
So the boron is happy even though it's only got those three bonds.
The question is, what's the shape?
We've been drawing these structures like this in 2D.
What's the real shape?
Now we can apply VSEPR because that's step one.
Step two is that I've got three bonding pairs, 3 BP, right, and no LP.
OK.
And so now if I have three electron domains, three electron domains, which I have, then the max separation for this case, max sep is going to be for them to spread out in a trigonal plane.
OK, so that's what they're going to do.
And that is called trigonal planar.
It has a name trigonal planar.
So you know, it's kind of-- planar.
So it's kind of right, but it's not quite right because I drew with 90 degree angles, and that's not what they are going to do to maximize their spacing here.
They're going to go at angles like that.
All right, they're going to find 120.
So if I have three electron regions, here we go, then-- now I had three-- OK, so the electron pair geometry.
Why is this different?
You will see in a minute.
The electron pair geometry covers BPs and LPs, but in this case, there's only BPs.
Fine.
There's still only three.
Right, and so it's trigonal planar.
Trigonal planar.
And guess what, if there is no LPs-- OK, so the structure that the molecule takes or those electron domains takes is trigonal planar.
The structure that the molecule takes if there is no LPs is also trigonal planar.
But now, the moment we've been waiting for is what happens when that's not true, and you've got a lone pair.
So again, we're going to do this by example.
OK, and so my next example is formaldehyde, which is something we love to talk about when we did Lewis.
So CH2O.
OK, so the Lewis structure for this looks like this.
Right, we drew this before, O, it's got the lone pairs out here, and then there's H and another H. That's formaldehyde.
OK, now hold on.
But there's no-- are there any lone pairs here?
There's no lone pairs.
There's no lone pairs.
So hold on.
If I go to two, I've got three bonding pairs and zero lone pairs.
It looks a whole lot like BF3, but it's different.
It's different, because now this matters.
Now this matters.
Right, and so now-- so now, I've got to add-- so it's like-- so 3 would give you trigonal planar.
Right, 3 gives you trigonal planar, because I've only got those three domains, but now I need four.
And there's four.
You knew there was something else, right?
Give more space to non-bonding domains and to bonding domains with higher bond order.
That's the fourth part of the recipe.
This just goes with what I wrote here.
Right, so I've got differences in the molecule between the BPs.
So you know now that I got to give more space to this double bond single bond repulsion than to these two single bonds.
All right.
So step four for this case is that the bond order is important.
And this molecule will bend.
So the shape of the molecule is going to be bent.
It will bend, because this-- I didn't draw it this way.
If I wanted to be right about it, I'm going to go like this, still not quite right.
Right, and this repulsion is stronger than that repulsion.
It bends the shape.
So it's not trigonal planar.
The electron domains give you a trigonal planar framework, but the molecule itself is bent.
OK.
And there's another example of that that we could do.
There's another example of that, and that is-- let's do it here.
And that is if we had the lone pair, which is what I mentioned before.
So I'm going to take another example here, which is SO2.
And in this case, uh-oh.
Am I making a mistake?
I can hear-- I can hear stuff.
Did I make a mistake?
No.
I see this.
I did.
I do, and that's wrong.
Bond order is important, but it is not under one lone pair.
It is not under one lone pair.
It would be under here.
And it would be-- if there is a bond order, it would be best if bond order.
Sorry about that.
Bond O. Trigonal planar, trigonal planar, slightly bent.
Look at this.
I should have just kept this.
Bent, bent, because now, I've got-- look at this.
In this Lewis structure, I've got two BP and one LP.
And finally, we have the case of the lone pair, and we can fill this column in, and it's bent.
And it's bent because, again, it's the same.
I go back to this as my key.
OK, so I did bone pair, bond pair, but there was a slight shape difference because of the ordering of the bond pairs.
Here, it's going to be even higher because I've got a lone pair.
Actually it's a lone pair double bond, but it's still stronger than a bond pair bond pair repulsion.
Lone pair bond pair, right in the middle is stronger than bond pair bond pair.
That's the rank order.
So this will be bent.
This will be bent.
So I've got three domains, and hold on, four LP more repulsive.
Oh boy, repulsive, and so that gives me bent.
Trigonal planar, trigonal planar, bent.
OK.
Did I get through that without making more mistakes?
So we're going to go further than this, which is why this is only the beginning.
But before I do, why does shape matter?
I told you shape mattered in the very beginning, but why does it matter?
Isn't it just important to know what the chemistry is and not the actual shape?
Why does the shape matter?
And so I thought I would give you an example of that with smell.
And yeah, right?
Exactly.
And actually, I think we should do this more often.
You know, I feel like this is inspiring.
We talk about stopping and smelling the flowers, but do we actually do it?
And look at that.
They've got one arm around the other.
This is a moment.
You can't share this moment on Instagram.
You have to put your phone down and be there to have this moment.
And I highly encourage you to be inspired by this.
Now, smell and taste are actually related.
And smell is, you know, it's actually a fascinating thing.
We can smell about 10,000 different smells.
It's remarkable.
A dog can smell between 10 and 100,000 times more.
Right, so if you do the analogy that people do with vision, we can see a third of a mile.
A dog can see about 3,000 miles.
Right, that's pretty cool.
But the question is why do we smell?
How do we smell?
How do we taste?
And it turns out that the way that we distinguish from one smell and one taste and another has to do with the shape of the molecule.
And so just like a key right, with a key, it's all of the same material.
It's the same chemistry in the key.
But the shape is different that can unlock the door or not.
That is literally how our receptor cells work for taste and smell.
This is a cartoon I found that I kind of like.
See, OK, shapes, circles, squares, right.
OK, and some shapes come in, and they make happiness, happiness, flowers and stuff.
I don't know.
Is cheese happy?
It looks neutral.
I would get happy with cheese.
But anyway, fish for some reason, but you know, it depends.
But it's shape dependent.
Now the thing is, taste works that way, too.
So this is what's happening in your tongue.
You've got these taste buds.
We've all heard the word taste bud, right?
But what is really going on?
What is really going on is a combination of chemistry and shape recognition.
So the taste bud, if you look at the taste bud, that's inside of the little pores inside your tongue.
There's a blow up of it.
And so this is what the surface of your tongue looks like.
And there's a little pore.
And there's little filters in there that helps certain molecules kind of get in there.
And what happens when they get in there.
Well, what happens is you've got these taste receptor cells that are like lock key pairs.
They actually only look at like the circle or the diamond, and they can tell you which one is which.
And that's a major part of how we distinguish from one shape to another, and it's actually so-- it goes back a long way.
Why?
Because shape and smell are literally survival.
They are literally like you can-- if you taste something, and it tastes poisonous, don't eat it.
You live.
Right?
So it is a very emotional thing to smell and taste, because it is actually coupled to your very survival.
And Democritus himself, Democritus, our friend Democritus, said that shape must be involved.
He thought that because things that taste bitter are sharp that the bitter molecules were sharp.
They must have sharpness to them, like shards of glass.
That's how he imagined.
And the sweet molecules were sort of the soft, fluffy spheres.
That's not that far off from being sort of what happens a little bit.
But when we look at it like, you know, glucose and quinine, we say, OK, those are very different chemistries.
So it might not be as obvious why one of them taste so different than the other.
But check out this example.
This is the same molecule, the carvone molecule that is called an enantiomer, which means that it has handedness.
It's the exact same chemical formula and the exact same structure, except for one is like this, and one is like that.
That's handedness.
Right.
And that difference makes one of them taste and smell like spearmint and the other like caraway seeds, right?
It's incredible.
It means that in our tongues in our noses we must have chiral, the ability to determine the chirality.
It's pretty cool.
Right.
So shape is critical, and this is one example of why.
This is one example of why.
OK.
Back to my VSEPR recipe.
Now, we've got to go a little further, because this is three electron domains, three electron domains.
They can be messed around.
They can be messed around.
Right, especially in this table, we're talking about lone pairs or not.
OK, I gave you the example, which I kind of fudged in there with bond order.
But what happens if I go to four?
So if I go to four, let's do an example with four-- if I go to four, I need some room here.
Why don't I do it in the center here?
Let's do an example of all possibilities with four, with four domains.
And there's three really good examples, right?
And you know them.
Let's do CH4.
So we'll do these kind of more quickly, CH4-- gesundheit-- which will be CH-- never leave enough room on top.
And H. There it is.
OK.
Anyway.
And NH3.
OK, so N H H H. And H20 to all O H H. This is how I drew it on the first slide.
Or this is how I drew it up there before.
Now the thing is that in each of these cases, what I want you to see in these three examples is their similarity first.
And their similarity is that if I pick my central atom, carbon, nitrogen, oxygen, if I pick my central atom, and I look around it, each one has four electron domains.
Right.
So in this sense, the kind of overarching electron geometry is identical for all three of those cases.
It's identical.
And it's tetrahedral, tetrahedral.
OK, that's the electron pair geometry that's exactly the same.
But I need to now count-- oh, there's the recipe.
I need to now classify the electron pair as bonding or non-bonding and then maximize the separation between domains while giving more space to non-bonding domains and bonding domains with higher bond order as we have now talked about.
So if I do that, here, what I have is four bonding pairs, zero, zero lone pairs.
Here, I've got three bonding pairs, one lone pair.
And now I really get to fill in stuff in my table, because now, I've got the examples of zero, one, and two lone pairs for the same electron domain cloud.
OK, so there is zero, one, and two.
You can see in methane, OK, the only way for this to maximize its repulsion, they're all the same bonding pair, they're all single bonds, so there's not going to be any kind of funny business of a double bond pushing harder, and there's no lone pairs.
And so this is just going to have the tetrahedral shape.
That will be the shape of the molecule.
That is the electron domain shape as well.
So in this case, it will be tetrahedral, tetrahedral.
OK, but see, now, in this case, I've got three bonding pairs and one lone pair, and we know because of the VSEPR recipe, which has-- oh, it's up there.
I keep forgetting it's up there.
OK we know that I want to maximize the separation, but I got to give more space to the non-bonding, because the lone pair bond repulsion is stronger.
Good.
So what that means is it's going to be sort of like tetrahedral, but in one place, there is no bond.
There's just this lone pair pushing down.
And so the shape that you're going to get is called trigonal, trigonal pyramidal.
OK, because now, that's just the way that shape looks.
So this is going to-- I'll show you actually a picture of this in a second, OK, of what this looks like.
And then with water, we now know that this will not be the most stable configuration, because these lone pairs are pushing on these bonds too much, so water is actually going to look like this, where these guys, the lone pairs can come out like that, right, that angles like that, be away from each other, right, and then the bonds are down there, and that's going to maximize the shape of the water-- I mean, it's going to minimize the repulsions that the electrons feel in the water.
And that is back to the same name, which is bent.
I've got a different number of electron domains, but I wind up with the same molecular shape.
See that?
Bent.
Now, I know this is kind of small.
I've got tables that have all this at the end that you'll have in the slides.
OK, so I know this is a little bit crammed in here, but the process of writing this is very, very fun and fulfilling.
Now, we can keep going.
But I want to tell you about a labeling system that's actually really helpful.
So if we keep going, we kind of want a system to be able to think about these things.
And so there is a system.
Again, this is chemistry.
We know how to name things.
Oh, but I promised you I'd show you that first.
So here it is.
There it is.
I didn't know why.
I click to that already.
That's this.
And the reason I wanted to show you this, this is from [? Avril, ?] your textbook that you're all reading so carefully.
And look at that.
This really brings it home.
Look at that lone pair.
Now you're seeing it as the electrons feel it, which is a probability distribution cloud.
That's the lone pair, and now you can see the lone pair wants space.
It wants room, and it's pushing down on these bonds, which are pushing away from each other.
And that's how you can figure out what shape it is, trigonal pyramidal.
That's trigonal pyramidal, OK, the molecule.
Right, as I said, we need a way to label things in chemistry.
So we can keep going-- linear, trigonal planar, tetrahedral, trigonal bipyramidal, oh, five, octahedral, six.
Don't get confused octa, eight, but octahedral is for six electron pair domains, right.
But we need a system to name this.
And so the chemists have brilliantly come up with AXE.
Now A is the central atom.
X is the bonding pair regions, and E is the lone pair regions.
X and E. OK, and so if I go back to like BF3, well, this would be-- oh there you go, AX3.
I say, what is the shape of AX3?
I know I've got to central atom, A, and I've got three bonding pairs around it and no lone pairs.
That's what that means.
I could go back as SO2.
Where is SO2?
Did I erase it?
Maybe I erased SO2.
I did erase SO2.
Oh, I didn't erase SO2.
Happiness.
Look at this.
SO2 would be AX2E.
Or if you want, you can put a 1 there.
It's a highly sophisticated, advanced labeling system.
That simply allows us to keep going, to keep going without writing so many words.
But we know now, A is the central atom.
X is the number of bonding pair regions.
E is the number of lone pair regions.
And we know about our rules, and we know about bond order.
So now if I look at those same-- really?
What happened to-- oh, there they are up there.
If I look at those same ones, well, OK, look those are tetrahedral.
OK, the electron pair distribution is tetrahedral, but-- gesundheit.
But as you can see in these pictures, you can get three different molecular shapes.
You can get tetrahedral, same as the electron pair.
You can get trigonal pyramidal, or you can get bent.
Those are the names of the molecule.
Notice that those aren't the names that include the lone pairs.
Lone pairs are how you find which one of these categories you're in.
But those are the names of the molecules.
Those are really important, those names of the molecular shape.
Now, if I move over one-- OK, oh there they are, names, tetrahedral, trigonal, pyramidal, bent.
Now, let's move over one.
So now I'm going to stop.
I'm not going to keep on writing everything down.
Let's see one last example.
So here's trigonal bipyramidal.
This is what happens when you have five electron pair domains.
Again, and I keep saying this, but I know that this can lead to confusion later.
So I keep on reiterating, the electron payer domain count, right, the five here, the five here, which gives us this overall kind of electron payer domain shape, that would be five there, right, trigonal bipyramidal, that includes a single, double, or triple bond is a domain.
It's a BP.
Right, it's a BP domain.
A lone pair is a domain.
How many of those do you have floating around your molecule, around your atom?
Sorry, your central atom.
And then you go down and you sort of decompose it in terms of how many of them are bonding pairs, X's, versus how many of them are lone pairs.
So let's do one last example.
And it'll be for five.
It'll be for five, right, so five.
And I want you to really-- I'll go with the center again.
I want you to really kind of get a feeling for why this works.
And this is, I think, a nice example.
If you had the molecule, if you had the molecule SF4, I'll give you two examples today that violate the octet rule, right, because chemistry lives in the fast lane.
If you have SF4, S is willing to have an expanded octet, all right.
As you go down in the periodic table, atoms are more willing to live in the fast lane and break the rules.
S-- we've drawn it like this, F, F, F, all right, we've drawn it like this, violating the octet rule and all that, but still being happy because it's lowest in energy.
And these are all my electron non-binding domains.
OK.
If I count-- remember, those aren't going to matter.
They're on the outside.
I'm picking one atom.
A is S. Right, A is S in this case.
And in this case, I've got-- you know, I've got, OK, how many body pairs?
I've got four bonding pairs and one lone pair, and one lone pair.
And so you can see-- I can just look this up here AX4E1.
It must be a seesaw structure.
But I want you to see it, and this is where playing with these models really helps you see it.
Because if you think about this, it seems, at first, that if I had four bonding pairs and one lone pair, why can't I just write it like this?
This seems like a good idea at first, right?
S, and then you've got a fluorine down here.
And then you're going to go out.
I'm using a kind of cool new notation here.
Right-- and then you're going to go.
Did somebody just say whoa?
Or is that just in my mind?
Like that, but I'm saying whoa.
That's a terrible rendition of this notation.
But this means-- now I've got to come on the board.
And for me, that's challenging because I'm not very good at drawing 3D things.
And so we use these little shaded in kind of sticks like that, and it comes out of the board, and then that's like the dashed one, and it goes into the board.
So there's one-- that looks terrible.
I'm going to try one more time.
So maybe if I just do this, F, F. And this is in the same plane here.
These three are in a plane, and that's going down.
And you've got your lone pair up here.
That looks pretty good.
Why is that better than something like this?
And now, one coming out of the board and the other going into the board, all right, and now this would be-- gesundheit.
This would be my other possibility.
I mean, if I think about, I think why can't it just be this?
These look-- I don't know.
These look nice and spread out.
Why not?
It's the lone pair again.
It's the lone pair.
It's got to be, because, you know, I put up there, somewhere, there's not there, that the lone pair repels the bonds more, and I've got more than say a bond and a bond.
But the point is I've got three of those here.
This has three, one, two, and three.
This has two.
This is a happier structure.
Right.
So I wanted you to see-- so this is the way to think about it.
You're using VSEPR.
You're applying these principles.
I mean, it's just a four-point recipe, but I'm hoping that in thinking about it this way and having the little models in your hand, which you have in this goody bag, that you guys will also kind of have a feeling for it, so that yes, you have the tables that are written out much better than how I wrote them out.
And here's one in the lecture notes that I'll leave you, right, total domains.
It's very similar, notation, structures, OK, shapes.
But also that you have a feeling for why this is the case.
All right, shapes of molecules.
See you guys on Wednesday-- no, Friday.