Flash and JavaScript are required for this feature.
Download the video from Internet Archive.
Description: This session explores how interactions between molecules weaker than ionic or covalent bonds give materials their properties.
Instructor: Jeffrey C. Grossman
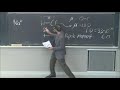
Lecture 14: Intermolecular ...
Today, we are talking about bonding between molecules.
Now, we have talked a lot about bonding within molecules.
Right.
And that got us to saying things like, well you can use electronegativity, You can use electronegativity, for example, to say, I got an ionic bond.
All right.
Or I got a non-polar covalent bond or I've got a polar covalent bond.
But today, what we're going to do is talk about how these molecules-- how these molecules bond together.
What sorts of interactions can they have?
And those are really important.
Right.
So that's going to be the topic.
Now, if we just get started with what we know what's up there.
All right.
Well, there's ionic.
So listen, I'm going to fill this in.
Bond, a model.
You'll see what I mean.
I'm going to draw a little cartoon.
The attraction, how it's attracted, the energy range, and an example.
Right.
So for an ionic bond, we've got ionic-- ionic.
OK.
And here we go.
Watch this.
Plus and minus-- there's my model.
OK.
And the attraction here-- we'll get a little specific.
It's a cation and an anion.
And we know that that's going to go like Coulomb's Law.
Right.
So the attraction, the energy, not the force, the energy will go like 1 over R, roughly.
Now, what is the energy?
Well, the energy is pretty wide ranging.
I'm going to do this in kilojoules per mole, since those are the units I have.
I'll write that in a second.
And an ionic bond can have lots and lots of range in here.
And it typically can be very strong.
Something we've already talked about.
An example would be sodium chloride, right there.
Let's put the units here.
The energy units are in-- I'm going to write it here-- kilojoules per mole.
Now, in a covalent bond-- so covalent wouldn't be here.
OK.
So in a covalent bond, remember, we've got something very different.
We've got this carrying a sharing kind of thing, and we've got maybe two atoms.
Right.
Two atoms like this.
And then the charge is sort of-- the negative charge is sort of floating around and sharing those two centers, like in H2 or Cl2.
I'm going to use Cl, so let's keep using Cl, all right.
That would also be an example of a covalent bond.
And the energy range there is also quite large, 150 to 1100 are the numbers I got.
The attraction is shared electrons-- shared pair of electrons.
And I'm not putting an R dependence, because for covalent bond, it's complicated.
It's complicated.
There is no.
It is.
It's a complicated-- but it's a very nice, happy relationship.
But it's a complicated R dependence.
So you can't simplify it like you can with a 1 over R or like some of the other interactions we'll talk about today.
OK.
1 over R, not for covalent.
All right.
Now that's where we've been.
Mm.
Mm.
Here we go.
Here we go.
Look at this.
I'm not even-- I'm just getting started.
Because the thing is, now, we got to talking about how these molecules can talk to each other.
And there's a variety of ways.
What I want to focus on first is this thing here.
That's that polar covalent bond that we've already talked about.
So we've already got a grounding for it.
Right.
But I want to remind you of what happens.
Right.
So if I have, let's keep with HCl.
If I have HCl, that's a nice version of a polar covalent bond.
And remember what happens there is you've got a sharing but sort of unfair.
Right.
So one of the atoms took a little more of the charge.
And so sometimes we represent that with like a more of the cloud, right, on one side than the other.
But it's still a covalent bond.
Yeah.
But the thing is that-- and so what you're left with like in this case is some positive charge on the hydrogen atom and some negative charge on the chlorine atom.
And we did this already.
We used these symbols.
And we also talked about how that leads you to a dipole moment.
What is a dipole?
Well, a dipole is just the description of what you have in this case, where you've got two charges of opposite signs separated by some distance.
OK.
Two charges.
Those aren't full charges.
Those are partial charges.
Right.
And they're separated by some distance, the distance between these two atoms.
And you get a dipole moment.
And it has a magnitude and a direction.
And remember, just to remind you, I did share this with you in a previous lecture.
But, you know, mu equals the charge times the distance between the charge.
Right.
So that would be like the charge would be the deltas here and the distance between them, right, would be this.
Right. 'r'.
Now, remember the units are in Debye.
So for this molecule, for here, mu is around 1.8D.
And 1D is equal to 3.3 times 10 to the minus 30th Coulomb meters.
Those are the units.
Charge times distance.
Charge times distance.
Right.
OK.
3.3 times 10 to the minus 30th Coulomb meter is a Debye.
And again, just to remind you, because we did talk about this when we talked about polar covalent bonds, the dipole moment of these molecules tends to range from 0-- mm-- right there.
Right, no dipole, to 10 or so-- 11.
Right.
Debyes.
Now, why is this important?
Because if you now think, well, what would happen?
What would happen if I brought another charge up to the dipole?
What would happen?
Right.
Let's say that I bring a positive charge.
Let's say that I'm a sodium and I've lost that outer electron, which we know is pretty easy to lose, if you're sodium.
Right.
So I'm positively in charged, and I'm coming along, and I see this dipole.
Well, it turns out that, as you can imagine, I'm going to be attracted to that negative charge.
Right.
So this molecule's going to orient in such a way that I can actually bond to the molecule and be attracted to the dipole of the molecule.
So that is actually another type of bond.
Right.
And we'll call that ion-dipole.
It's an ion-dipole bond.
OK.
Let's do this so that I stay within the lines.
All right.
Now, OK.
So what does that look like?
Well, we just said it's a charge.
I'm taking a single charge, and I'm bringing it to this thing that has a shift of the charge within it that led to these two partial charges.
So, as you as you can imagine, it's going to want to orient to form a bond.
All right, so let's draw it that way.
All right.
So maybe if I were to take HCl, maybe I would have sort of like, you know, a cloud like that.
Right.
Where it had it's a minus and it's plus there.
OK.
Why did I-- I don't know why used dash lines, but there you go.
And so you can see that is-- what I wanted to put is the dashed line for the bond itself.
Give myself a little space.
Right.
That's the bond we're talking about.
That's the bond we're talking-- Now, it turns out that this is an important type of interaction between molecules and ions.
It's an ion dipole interaction.
And it has a range-- I wrote it down.
It has a range of 40 to 600, huge range, 40 to 600.
And the kind of attraction is really not describable in any other way than what we just described.
It's an ion and a dipole.
And it goes as 1 over r squared.
If you look at the separation, the distance between these, right, that distance-- the dependence on the energy, on the bond energy, will go like that distance, like, 1 over that distance squared.
Now, these dependencies you can get from fairly simple electrostatics.
I won't derive any of it.
I'm telling you what it is.
OK.
Good.
So an ion double-- now an example of that would be the system that I just drew.
Right.
So Na+ plus and HCl.
OK.
Good.
That's a different kind of bond.
But see, now, I could also have two dipoles.
And I got a picture for that.
So here's a dipole.
There's my HCl dipole, and there it is with the negative partial charge and a positive partial charge.
And it's also color coded.
Right.
OK.
So that's cool.
And there they are just floating along.
And I put an electric field on.
And I put an electric field on.
And you can see that with the electric field-- so there's my positive plate and my negative plate-- they're going to try to align.
Right.
These dipoles mean that they're going to align with the field.
They're going to align with the field.
So all of those positive edges are going to try to turn and face the negative one.
And all those negative ones are going to turn and face the positive one.
And that's exactly what we just did with this ion.
We basically did the same thing here.
That's what the ion did.
OK.
That's what the ion did.
It said, hey, come and face me and let's form a bond.
But these can also be attracted to each other.
All right.
And so I've got a picture there.
So you can see, like, if I have a dipole in another dipole-- so this is not an external field, and it's not an ion.
It's two dipoles, two HCl molecules.
Right.
Well, they each have a plus charge and a minus charge.
They each have a plus charge and a minus charge and, you know, partial charge, right, polar covalent.
And you can see that those can line up, actually, in different ways to form attraction.
And they can also repel.
Right.
But overall, when they get together, or when two of them get together, they're going to find a way to attract, because they can-- because they can.
And so that is another kind of inter-molecular attraction, and it's called a dipole-dipole.
Dipole-dipole.
But, let's draw this.
And if we do that we have-- let's see.
I'm going to try to draw this.
So I've got one dipole.
I'm going to stick to that picture-- oh, I'm going to stick to this picture, but it's the same as this picture, right, where I'm actually using the kind of shape here to show you that more charge has gone over to one side.
OK.
So let's go back to here.
Right.
And so if that happens, then I've got minus and plus, thank you.
OK.
And then I'm going to have another one that's going to want to line up like this.
Oh.
Did I do that right?
Yeah.
And this is the bond.
This dashed line here is the kind of bond that we're talking about.
Yeah, those are almost sort of asymmetric.
OK.
Now-- gesundheit.
Now, that's a dipole-dipole.
We don't really have a better way of calling that either.
They're dipole charges interacting with dipole charges.
And that's going to give you a 1 over r cubed dependence.
And, you know, if you've taken some basic ENM or if you do, you'll learn this.
Right.
You'll learn how to derive these distance dependencies.
So I've got two dipoles, right, because there are two molecules that have not-- is not a non-polar.
It's a polar covalent bond.
And those are attracted to each other in this way.
And the range of that bonding strength for two dipoles is 5 to 25.
So it's a lot less.
Right.
And well, if you had HCl and HCl, that would be an example-- now instead of an ion, I'm putting two polar molecules in.
Right.
So this could happen.
This could happen from a molecule to a molecule.
It could happen from an atom to a molecule.
It could happen from a molecule to an atom.
Right.
So for example, you know, I could have-- if I have HCl, and more charge has gone to the chlorine side.
Right.
So this has my delta plus.
This is what I wrote over on the other side.
That's my delta minus.
And if I have just a single atom-- I'm going to put a xenon atom here.
Well, it's just sitting around happily, you know, minding it's own business.
And what's going to happen to that?
Right.
What's going to happen to that?
Well, see, if this comes up to an atom that didn't have a dipole, it still has feelings.
It still has feelings.
Why?
Because electrons have feelings.
That's why.
Because it has electrons.
So those electrons are in there, and it doesn't have a dipole.
But that's OK, because those electrons still can feel.
They can feel the dipole of this.
They can feel the dipole of this molecule.
And when they feel that dipole, they react to it.
They react to it.
So there's a whole other thing that can happen.
By the way, it could happen-- it could happen with a sodium atom.
Na+ also is a charge that this happy go lucky xenon, it doesn't know what's coming.
But it's got electrons that feel charge.
And so you bring in charge to it, and they're going to react to it.
They're going to react to it.
Whether it's a single charge or whether it's a dipole, these are charges that are going to change the charge around that atom.
Now, there's another name for that.
And that leads to another kind of bond.
It actually leads to another kind of bond.
Let's see.
So I'm going to just-- let me draw the cartoon.
I really want to make sure you understand this, because this sets up the next kind of bonding.
And so let's suppose I take a sodium, and I'll use H2.
H-- gesundheit.
H. And mine could have been a single atom.
It could be a non-polar molecule.
Right.
So this is what this looks like.
And now this is going to-- these are kind of walking along happily, and then they see each other.
And all of a sudden, this is going to induce a shift of the charge around that H2 molecule.
So what you're going to see is now more-- which way is it going to go?
Which should have the bigger side, the more charge?
Right, yeah, here.
Because that's positive, and electrons are negative.
So now it's going to look like this.
Now, that was not a dipole.
It is not a molecule with a dipole.
But it's an induced dipole, induced dipole, because of feelings.
It comes down to feelings.
That has a name.
All right.
That's ion.
that one right there is ion-induced-- see if I can fit it all here-- induced dipole.
Ion-induced dipole.
And I'm going to try to draw this now.
Let's see.
OK.
Hang on.
OK.
So the ion-induced dipole-- I'm going to have a plus charge here, and here's what I'm going to do.
I'm going to draw the original shape as what it was.
Oh, boy.
Here we go.
That's what it was.
But then it got induced.
OK.
It got induced.
And so then, once it's induced, you've got like a minus and a plus.
Happens all the time.
It happens all the time.
Now, the way that it will-- we'll talk about this in a little bit of a different way, because this attraction is between an ion-- by the way, an ion just means an atom or something lost a charge or has a charge, plus or minus.
Remember, cation anion.
But the ion in this case is interacting with what is called a polarizable-- polarizable electron cloud.
Now, the electron cloud is nothing new.
We got an electron cloud nailed.
We know about electron cloud.
Right.
Because those are orbitals.
But what is this thing called polarizable?
Right, polarizable.
And that's a very important term.
So I'm going to write down its definition.
Because you can imagine if I have a way of being attracted to a non-polar molecule by shifting its charge, by shifting its cloud, then how easy was that?
How much could I shift it?
You can tell that that's going to affect the bond strength.
By the way, which is-- let's see-- 3 to 15 and has a 1 over r to the fourth dependence.
And let's give us-- let's give the example that I gave there.
Na+ and H, H.
OK, non-polar molecule.
OK.
It could have been an atom.
But It could have been a non-polar molecule.
Yeah.
Now, polarizable-- polarizability, is a very important concept.
Polarizability is the measure-- gesundheit-- Of how easy it is to temporarily distort the electron distribution.
That is a very important concept for these types of bonds and for a lot of other properties and, of course, for life in general.
How polarizable are my electrons?
I need to know.
Are they just willing to deform from their happy orbitals?
Temporarily, because if they were going to deform permanently, then you'd have a dipole, a permanent dipole.
These are induced dipoles.
OK.
That's important.
OK.
Now, OK, good.
So that's an ion-induced dipole.
Now you could also imagine, you know, this could have easily been a dipole over here.
It didn't have to be an ion.
It could have been a dipole.
I could have had a dipole here that came up to a non-polar molecule or an atom, right, and it could have induced the same shift in the charge density, which then allows me to be attracted, because there's a little bit of a fluctuation of negative charge there.
Right.
So if I were to-- so the thing is, we've been talking about dimers.
But you could talk about, what is the dipole of something more than a dimer.
So this is polar.
What about these other things?
Right.
So if I look at molecules, right, that are polar, it's not just about HCl, right, which is that first one up there.
But that's kind of the obvious case.
But what about CCl4?
What if I take a carbon atom and I put 4 chlorines?
Those are all polar bonds.
And you can see-- well, it's hard to see-- but they've drawn in the dipole moment.
You've lost some charge from the carbon.
It's gone out to the chlorine atom.
There's a dipole movement within each bond.
But now here's where VSEPR comes in, because these are going to be tetrahedrally distributed and fully canceling out.
So it's like you've got all these kind of electrostatic things going on that all cancel out.
So this molecule is not a polar molecule.
Right.
On the other hand, molecules NH3 or CH3Cl-- right.
Now, this is going to be polar, because these dipoles do not cancel out within the molecules.
So you can kind of use this thinking.
There's BF3, trigonal planar.
Right.
All those dipoles cancel.
It's a non-polar molecule.
But if you take a classic example-- which we'll come back-- of, say, water as a polar molecule, I could do the same thing.
Right.
I can take water, which is oxygen, hydrogen, hydrogen. And you see you've got delta plus there, delta plus there, and you've got some minus there.
Right.
Now, there's a dipole moment, so part of it cancels but not all of it.
So there is a dipole moment for the net water molecule.
The net dipole is what matters to determine if a molecule's polar or not.
So there's a net dipole, net dipole when you sum them all up.
And that's what's going to determine if this can come along to that xenon atom, right.
And so here's my xenon just sitting there doing nothing.
And now when water comes up to xenon, what's going to happen?
Well, what's going to happen is I've got my water molecule, my water molecule, my delta minus, my delta plus, my delta plus.
And because it's got a net dipole, it's going to form the atom.
And so I'm going to have-- let's see, make sure I draw this right.
So it's going to look kind of like this.
Delta minus, delta plus, and that allows a water molecule to bond to a xenon atom.
It is bonding with another bond, which is very similar to what I just wrote-- gesundheit-- But it is a dipole-induced, not ion.
It is a dipole-induced dipole.
You see that?
Because now, I didn't have an ion.
I had a water molecule that had a dipole moment.
So now I've got my dipole-induced dipole, and that's going to look-- oh let's draw it.
Let's see.
So I got my dipole-induced dipole.
So we're going to go, kind of, oh, boy, there we go.
OK.
Minus, plus, and then-- now that might be the dipole of, say, the water molecule or something that.
And then I've got another thing that didn't have a dipole, but it got induced to have one.
OK.
Oh, boy.
I went out of the lines.
Ah.
I was so careful.
But you can't-- you can't hold chemistry.
Uh-uh.
No way.
No way.
Mm.
OK.
Delta plus, delta minus, it's induced.
That's what that means, right.
Now, it turns out that this has an even weaker-- so this would be a dipole.
Let's just-- right, let's finish this up-- dipole interacting with the polarizable electron cloud.
And the value of this is even lower range, 2 to 10, and the dependence is 1 over r to the sixth.
And the example-- let's go back to HCl.
HCl, there's my dipole coming in ClCl.
I like that because it's got three Cls.
Why not?
That's a non-polar molecule.
That's a dipole.
That induces a dipole in this by shifting its polarized electrons around.
Right.
And then it allows there to be a little bit of attraction.
You might be saying, but that's so little.
We'll get to that in a second.
That's not so little.
That's actually not so little when you've got a lot of it.
OK.
OK.
Dipole interaction-- now it turns out that even-- and this is what's mind blowing.
You knew this was coming.
This moment today was going to come.
I can have an interaction without any dipole to start with.
It's true.
I can take two non-polar covalent bonds, two non-polar covalent molecules, H2 and H2, and they are attracted to each other.
How is that possible?
Well, it's what explains this.
I mean, we know-- why does helium, argon, and xenon-- why do these have different boiling points?
By the way, the boiling point of these-- all right, so these can be made into liquids and you can boil them, right-- is really different.
And it's a measure of how strongly they're bonded together.
Right.
So this is a direct kind of way of thinking about, of measuring something that's related to the bond strength.
So why do these atoms-- they're all just atoms-- you know, they're all just like this.
They don't have this dipole.
Right.
They're non-polar.
They're just atoms sitting there with their symmetric charge clouds.
How is it that they can be attracted to each other in the first place?
And how is it that they can have such a strong trend?
And that has to do with another kind of force.
And there is a picture.
So here's two examples.
There's two helium atoms.
So that's the first one in this list, boiling point minus 269 degrees Celsius.
It's pretty cold.
And there's two non-polar molecules, H2.
What happens is that you get fluctuations.
You get fluctuations.
And what I mean by that is that, just randomly, literally, randomly, right, because of collisions or thermal energy-- randomly, one of those could form a dipole.
I didn't mean it.
It wasn't my happy place, but it happened.
Now, if it happens and your charge kind of goes to one side, even if it's just for a split second, that's a dipole that then, if you're near another atom, can induce a dipole.
And we know that if we can induce a dipole, if we're here, if we're at this stage, we've already got that covered.
We already know that I can induce another dipole if I'm charged.
Right.
And so what happens is you get this kind of fluctuation that leads literally to a bond.
It leads to a bond.
Right.
And that is called London dispersion.
That's called the London force, and the London dispersion is the name of a kind of bonding you have.
Let me just make sure-- ha.
OK.
London dispersion.
Notice there's room for one more.
It's coming.
There's more.
We're not done.
We are not done.
I can literally take two non-polar atoms or molecules, and I can find a way for them to be attracted.
And the way it happens is thermal fluctuations.
This is such an important force, and it's such a strange one that I want to write down what it is.
It's that non-polar-- we've been talking about polar and charges, but non-polar molecules are attracted by fluctuations.
Fluctuations.
I should say, by dipole fluctuations.
That's really what's happening.
Right.
By dipole fluctuations.
So one of them gets a little shift, and there's a dipole.
And then that dipole, if there's another one around, can induce a shift, and then there can be a bond.
And now, this gets back to something very important, which is that-- you can imagine, how easy was it for temperature-- how easy was it to create that shift?
Right.
Well that's going to have to do with your polarizability.
Now, OK, so what is it that has to do with polarizability?
Right.
Well, if-- oh, I do have a board here.
Ha.
OK.
You can already imagine, if I go back to this list here-- if I go from helium to argon, what have I done?
I've gone down in the periodic table.
And we've already talked about this.
Those electrons that are going to do the shifting to cause the fluctuation-- those electrons are more loosely bound.
Right.
They're further out.
They're more loosely bound.
We've already talked about this.
We've looked at this in a lot of ways.
Right.
Whether it's radius or ionization energy, those are-- if they're more loosely bound, then they're easier to shift around.
I'm not taking them out.
Right.
I'm not taking them out.
But I'm moving them.
That's what London is.
I got to fluctuate it.
So you can imagine now, why, if it's heavier, and those electrons are further out, they're more polarizable.
Right.
So let's write that down.
Right.
So the London-- so we're going to get a stronger London-- two reasons.
One, heavier atom, which really, in this case, right, it's heavier, but it really just means more polarizable.
If it's more polarizable, that's the key.
That's the chemistry key, more polarizable, more polarizable electron cloud.
Write it all out.
But the other thing that you can imagine with London-- right, so that explains that trend.
The other thing that you can imagine with London is that these interactions, these fluctuations, they're happening all over the place.
And the more contact I have-- the more places in a molecule I have to induce this, to have this fluctuation happen, the stronger these forces could be.
And this is exactly what we see.
Look at this trend.
Methane, ethane, propane, and butane.
All I've done is I've added another carbon atom.
Oh, we're going to get to these.
We'll talk a little bit about Orgel later.
But for now, look at the boiling points of those.
Again, boiling point being a proxy for the bonding strength.
What bond could they have?
None of those, only this.
Ha.
I got excited.
Only in London, because look, they're non-polar molecules.
They've got no charge on them.
This is the only way they can talk to each other.
But look at how much they talk to each other.
Now, why?
Because of the second thing, right.
Which is that the greater the surface, the greater the surface.
You can have more.
The more surface you have-- the greater surface, more contact area, more contact area.
And that's how this bond-- that's how this thing works.
Because as long as I've got more surfaces see each other, then there can be more fluctuations, right, that are happening, that then induce the other one to have a fluctuation, that then create a little moment of bonding, that dramatically changes the properties.
And look at this.
This is a little bit small, but, you know, these are the-- so let's go all the way, right.
CH4, OK.
Let's go all the way, and look at this.
It determines when you have a gas at room temperature or when you have a liquid at room temperature.
Boy, is that important for how we use these molecules.
Right.
Boy, is that important.
It's all London.
there's no other option.
Right.
But that's what's dictating this trend.
It's even more mind-blowing than that, because look at this.
This is one molecule that's identical.
This is C5.
This is 5 atoms of carbon, n-Pentane.
Same exact grams per mole, because it's the same chemical formula.
But look here, 36.1 degrees C is the boiling point, and here it's 9.5.
Why?
It's all this.
This is not the ground state.
The most stable structure is here.
But there is a version that's called neopentane.
It's like a nickname for it.
There's a version of it that you can make that has a different shape.
And because this shape cannot come into contact with itself, look at this, you can see it from the red line.
It's coming into contact with itself.
In the liquid or gas, it can't have as much London forces, London potential.
So it cannot bind as strongly, and therefore the boiling point is a lot lower.
It's a lot lower.
Now, there's a little bit of naming confusion.
I want to clear this up.
Oh, boy.
I have nowhere to go here.
So I'm going to get rid of London for a second.
And I want to make something very clear that there's another word that's used, which is called Van der Waals.
OK.
Now, this ability of a fluctuation to happen, which then induces a fluctuation, that can happen no matter what molecule you have.
It can happen.
And it does.
It's always there.
All right.
So all molecules, whether they are polar or non-polar-- polar or non-polar, all molecules are attracted by London plus any other attractive forces-- any other attractive forces present-- forces present.
OK.
So London is always there.
Now, people interchangeably use the term Van der Waals.
And what Van der Waals encompasses is more than London.
Van der Waals, what you will sometimes see in textbooks, is the Van der Waals force.
OK.
So that is any or all added together.
This is the combination of these weak forces of dipole-dipole-- dipole-dipole.
Let's see, dipole-induced dipole, and London.
Those taken together you will sometimes see referred to as Van der Waals forces, or weak forces.
But we know more than that.
We know the distinction.
Right.
We know that London started like this and then went like this.
Ah!
Really bad.
Gesundheit.
Right.
We know that it started with non-polar, and that there's a difference.
And so this is all about the polarizable electron cloud.
Polarizable electron cloud, that's all you got.
Right.
And this can be really small, 0.5 to 40.
Oh, yeah.
Let's just keep with chlorine 2, chlorine 2.
Two non-polar molecules, London dispersion, and this also has a dependence of-- in terms of the distance-- of 1 over r to the sixth.
Now, in case you think that's small, I want to show you a little video of why this matters.
So weak forces-- now, weak forces are really strong.
We know this because of "Mission: Impossible".
We know this also because of our own students.
There was one of my former students, Rory, and he won all sorts of prizes, because he was trying to be like a gecko.
And so he made gloves that were like gecko gloves, and much to the delight of the facilities people at MIT, he actually climbed one of the buildings using his gloves.
In case you are wondering, it wasn't actually their delight.
But really cool stuff.
So let me show you.
This is like a 30-second video.
He found the answer in the sheer number and design of the hairs on the geckos feet.
Geckos have millions of microscopic hairs on their toes.
And of course, we can't see this with our naked eye, because each hair is only 1/10 the size of a human hair.
And each of those hairs branch down to billions of little split ends.
And they can make such close contact with the surface that weak intermolecular forces can begin to add up to something really strong.
Turns out geckos exploit something called the Van der Waals force.
No.
London, London.
If you think of an atom as a dancing couple, when you bring two atoms into very close contact, part of one atom can get attracted to part of the other.
That very weak bond is the Van der Waals force, and it sticks atoms together.
Proximity is the key.
But bringing two materials that near each other is harder than you'd think.
All right.
Now, obviously, that got me very excited when I saw that, because that is how I see atoms, and that is how I see electrons.
And I saw this, and I almost fell over.
And of course, I played it, and I know all those moves.
I would suggest you guys-- it's a Friday.
There's some good moves in there.
There's some good moves in there.
And you can take this on your phone and just kind of work it out at the club.
And speaking of the club, there's one more thing I've got to teach you.
Because it's that last line.
It's that last line.
See, if you go along a trend like methane, silane, germane, right, these are non-polar molecules, and they're all attracted to each other by these Van der Waals.
And you can see that the boiling point, remember, our proxy for the force, the bonding strength, is going up.
That's what you'd expect.
That's because these are getting larger, and there are going to be more polarizable electrons.
But look at what happens as you go to other molecules.
This is the expected trend until you get here.
Right.
NH3-- and that is because of one last type of bond.
That is because of something called the hydrogen bond.
It's a very unique kind of bond.
And so a hydrogen bond is our last intermolecular bond.
Hydrogen bond, right.
And it's a very particular kind of bond.
And it has to do is something very particular that hydrogen does.
Look at those boiling points.
Look at the change.
I mean, H2O is H2O because of the hydrogen bonds.
Right.
Earth's operating conditions are luckily right here.
But this is only good because of hydrogen bonds, and it's all the way up and not all the way down there.
So hydrogen bonds are unique.
This is the electronegativity scale, and there's something very important that happens for those three elements.
Those are the three elements that are in this trend.
Those are the three elements.
See, nitrogen, chlorine, and oxygen, those are the three elements here.
And what's important about them is that they have such a huge electronegativity.
And so in a hydrogen bond, what's going to happen-- what's going to happen in a hydrogen is they're really going to pull a lot of charge from the hydrogen.
So you have a very high positive charge on the hydrogen, but there's something else that happens.
For hydrogen bonding to occur, you see, it's the H with say fluorine, and you have a delta plus and a delta minus, and this is high.
This is very high.
All right.
But you also have a lone pair somewhere.
You've also got a lone pair somewhere.
And we know that we have that on the fluorine, right.
We know that, and we've got those two.
And the bond that a hydrogen bond represents is the fact that I've done two things with the same molecule.
I've taken a lot of charge off of hydrogen and created a very strong delta plus on the hydrogen.
But I've also got a lone pair somewhere that that can bond to.
And when I have those two things, which I really have for these three elements, right.
When I have those two things, I can make this very special kind of bond.
Now, this is what it looks like.
Right.
So there's an H2O molecule, and I want to make a point here.
There's the covalent bond between the oxygen and hydrogen. There's the delta plus.
And there's that hydrogen bond right there.
Mm.
Mm.
There.
OK.
Hydrogen bond that forms between the delta plus and the lone pair.
Now, you can see why water is so special.
You can see.
Because unlike NH3 or HF, you can see where water had the biggest kick up from what would be expected.
Because unlike NH3 or HF, you have exactly the right balance.
I've created two of these very strong delta pluses, and I've got two lone pairs.
It all all works out.
There's no extra lone pairs, like here.
And all these extra lone pairs, right, that don't-- you know, each molecule only has one hydrogen. Here, it's two and two.
That's why water is so special.
This bond is so important.
I know you've probably heard about it for water, but here's the example I want to give you for the club, because some of you might be able to drink ethanol, only if you're at the right age, please, of course.
This is what's in alcohol, right.
This is the ethanol molecule.
And it has a boiling point of 78.5 degrees.
Again, that tells you something about the bond strength of this molecule to another molecule.
But look at this.
That's an oxygen with a hydrogen.
This took a lot of charge from the hydrogen, right, so you've got a strong delta plus.
But you've got those two lone pairs there ready to bond to the other ethanol molecule.
But look what happens.
Methoxymethane is a gas at room temp.
It's boiled off.
It's the exact same chemical formula.
It's the exact same chemical formula.
All I've done is shift the oxygen in, and so now there's no hydrogen that's had that big of a delta on it.
So it cannot hydrogen bond.
It can London.
You know.
But it can't hydrogen. It can't.
Because the oxygen has the lone pairs.
But there's no hydrogens that have that strong of a delta.
So when you're at the club tonight, trying out those moves, go up to somebody and say, it's a really good thing that's not methoxymethane, isn't it.
Really good thing.
And tell them about hydrogen bonds.
Have a great weekend.