Flash and JavaScript are required for this feature.
Download the video from Internet Archive.
Description: Continuation of Lewis structures, covalent bonds, and resonance.
Instructor: Jeffrey C. Grossman
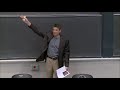
Lecture 10: Lewis Structure...
OK now, exam one.
I mentioned this before.
I thought this would be helpful to everyone.
What I did is put together what I call a concept map.
And I will post this, here it is, oh, there is a periodic table, isn't that lovely?
This is what I put together for you guys.
And I call it exam one concept map.
Now, I'm not going to go through it now.
But I wanted you guys to have the big picture of what we've done.
This really is a celebration, especially when you look at all of the things that we've learned and how they're all connected together.
And that's what I wanted you guys to see.
OK so, look at this.
So here are on the left here are-- this is all not graded stuff, here's what's been graded.
Oh, and this is what's graded on Monday, exam one problem topics.
Look at all those things.
Now over here, we have the three goody bags that you guys have had.
And there have been problems in there.
And here we have the problems that you've had from the textbooks and the topics there.
Here we've had your seven recitations.
Here you've had-- it's even color coded.
It's even color coded.
So here you've got the lectures.
So these the nine lectures and all the topics that we've talked about.
Today is number 10.
And as I said, I will tell you something today about Lewis structures that will not be on the exam, and that is resonant Lewis structures.
So we'll teach that.
And then those are the three quizzes.
Now you notice, there's some things, like here, OK, we've covered this stuff, like Lewis dots, and electronegativity, Lewis structures, stuff like that we've covered that has not been on a quiz.
But it could be on the exam.
So this is a concept map that I hope gives you a sense of how this all connects together.
And how this connects together to give you the knowledge that you need to answer questions about these topics.
Now, the exam is not-- these exams walk the balance.
We want you to learn how to solve these problems.
What I want you to do on Monday is run out of knowledge, not run out of time.
Did I get a-- thank you.
Now, you might run out of both, but listen-- [LAUGHTER]
But these are not meant to break you in a speed test.
These are meant to see if you have learned the concepts.
So what I ask you to do on this exam is show us what you know.
As you may know already, I am a big fan of partial credit.
Lots of partial credit.
If you show me you know something, you will get credit.
And so that gets into, also, exam strategies, but a lot of you already know about this stuff.
But please remember, don't just go in order, and then run out of time, and not even see the last two questions.
Take a look.
Tell me what you know.
Show us what you know.
We're not about the last decimal on some calculation.
I'm much more about do you understand the concept?
Do you know how to solve this problem?
So show us that, please, on the exam.
OK, now was that another loud snap in favor.
I think that means it's like positive reinforcement.
Topics, OK so, the other thing I want to say about the exam is we're going to connect concepts together sometimes.
That's the point.
Exams aren't just going to be another three quizzes plus one more question on Lewis.
No, we're going to see if you can also weave together the knowledge.
So an example would be, if I were to ask you, OK, I'm going to give you a PES.
So let's see, here would be like a question about a PES question.
So here's a PES diagram.
And here I'm going to draw, I'm going to say, OK, I give you this PES.
Here we go, let's label it, three, four, five, six.
We don't need more than that.
OK so, we're going to go, oh, and a break in the axis.
Now you know all about that.
And we're going to go all the way up to six here.
And we'll do another break.
We'll go up to two there.
And now this is going to go up to here.
And you can read off of here.
Remember, with the PES, that's a relative electron count.
I'm blasting this atom with enough photon energy to get all the electrons out.
And then I'm counting their relative-- I measure the kinetic energy, I can get the energy.
Oh by the way, this would be like decreasing, the way I plotted it before.
And the way we usually plot PES diagrams is the x-axis is going down in energy.
Because those are the 1S electrons there.
These would be the 2S.
And these would be the 2P.
And I can keep on going.
So I've got 3S.
Now what do we got here?
We've got 3P, How many?
Four.
OK, good.
OK, a question you might be asked, what atom is that?
Sulfur.
OK, good.
OK that's sulfur.
So I can answer that kind of question.
But now, let's take another concept and weave it in here.
OK, if that's sulfur and I say, well, what if I went up to chlorine.
Right so, sulfur has 16.
Sulfur has 16.
And chlorine is 17.
What would this look like for chlorine?
I gave you this.
Well, you might say, well, I know, it's going to just be like this.
But let's go to the right height.
And now I filled that with the one more electron.
And so it must be chlorine.
But that's not taking everything you know into account.
Because you know that if I go up to chlorine, I've added a proton.
But I haven't really done much more to shield those outer electrons.
And so everything wants to be closer to that more positive charge.
So you've got a reflect that in the PES.
So if I were really going to say, well now, you know.
You might not even know quantitatively.
But you know qualitatively, if I'm going to draw this peak for chlorine, it better be to the left of that peak.
It's got to move in just like the orbitals and all those things we've talked about.
That's the kind of thing that I mean.
I want you to be able to synthesize concepts as we think about exam questions.
Everything is going to shift over, if you go to chlorine.
Now, on Wednesday, we talked about Lewis structures.
So let's talk about Lewis structures more.
Let's suppose I've got acetaldehyde, a wonderful toxic compound, H4O.
OK, here is a possible Lewis structure for this.
All right, let's see, I'm going to draw the two carbons here.
Here's my oxygen. It's got some lone pairs, put another one there, why not.
Hydrogen here, and we got one more hydrogen there.
What is wrong with this?
Double bond on the hydrogen. Question really is, what is not wrong with this?
I can count three things, right now, that are wrong with this.
What are they?
Double bond-- this has to have, remember, these things need to be happy and follow their octet.
Octet means for hydrogen, two.
But otherwise, so that's good.
That's good.
That's not good.
OK so, we're not-- is this good?
No, no.
Is that good?
Now, hold on, because OK, but there's something else that's fundamentally wrong with this.
Anybody notice?
How many electrons?
This is how you start a Lewis problem.
How many electrons do I have?
How many electrons do I have?
Remember Lewis is all about the valence.
So I've got 4 times 2, that's 8.
Eight electrons, four electrons here, and six electrons here, how many do I have?
So I've got 18 electrons total.
Now what did I put into this diagram?
So each of these bonds, remember, a line is two electrons.
It's a bonding pair.
So I've got 2, 4, 6, 8, 10, 12, 14, 16, 18, 20.
I didn't even get the electron count right.
I didn't say it's acetaldehyde two minus.
Wrong number of electrons.
And octets not formed.
There's something else.
There's another way you can see how this Lewis structure is wrong.
How is that?
What else did we learn on Wednesday?
Really important concept.
Formal charge.
Formal charges are all over the place.
Lowest not lowest formal charge.
Now, when I say lowest, I mean closest to zero, because that is what gives you the most stable structure, as we talked about on Wednesday.
You can see this, formal charges here are all over the place.
So the formal charge on this carbon is-- OK so, you go the number of valence electrons of the free atom minus dots minus 6.
So 4 minus 1, 2, 3, so that's plus 1.
Oh, 6 minus 1, 2, 3, 4, 5, 6, 7.
So that would be minus 1.
So this is not a good Lewis structure for all of these reasons.
The right Lewis structure would look something like this.
Very easy fix.
And C, and here you go.
And now, you can check all of these things that we just talked about.
You can check the formal charge.
You can check the total charge.
I could've taken two electrons off of here.
And it still would have been wrong.
But this is the right Lewis structure, because we satisfy all the things that we talked about on Wednesday.
OK, good, now, as I mentioned, there is one other concept oh-- The last concept that will not be on the exam, oh, but it could be on exam two, I don't know, is the last concept about Lewis structures that's very important.
And it's what happens when you have structures that look similar.
If I look at all these things, number of electrons, octets forming, formal charges, these look good.
These both look good.
And so the question is, which one of them is right?
And this gets into this concept that I want to teach you, which is called resonance.
And just to define resonance, I'll put that on the board.
These are called resonance structures.
OK now, resonance, when referring to Lewis structures, the way I want you to think about it is that it describes delocalization.
I'm going all caps, delocalization, why not?
I'm not shouting it.
I'm just saying it's an important concept.
Describes delocalization of electrons in molecules.
So in the case of ozone, which is something we broke apart and talked about, we talked about ozone already, in the case of ozone, now, what's happening?
Well if I draw the ozone molecule the way-- if I draw it the way one of those is, so I'll draw it the way the left hand picture is, like this.
And O and, I'm not talking about shape.
No shape yet.
[INAUDIBLE] I don't know.
Next week, shape.
This week, no shape.
OK, hold on.
Now, if I look at this molecule, this is a double bond.
There's more electrons on this bond than here.
And what we know about that is that that's going to make that bond stronger.
And those atoms are going to be closer.
So this length would actually be around 1.2 angstroms.
And this one would be around 1.5ish.
But see, and now, you say, well, maybe it's the other one.
1.2 over here 1.5 over there.
But now, it take ozone, and I can measure it.
I can measure those bond lengths.
And I do that.
And when I do that, what I find is that it's neither of these.
But in experiment, the bond length is 1.3.
And it's the same.
It's not different.
So what is happening?
Physically, what is happening is delocalization.
And remember, electrons do things to be happy.
Happiness means lowering the energy of the whole system.
And so what these electrons realize, what these extra two electrons here realize is well, OK, I couldn't make this bond short and that bond long.
But maybe if I delocalize across the whole molecule, maybe if I do that, I can be happier.
Meaning I can be in a lower energy state.
And that is what resonance talks about.
That is what it tells us about.
It tells us when I have these different states that look equivalent, that the molecule essentially, pictorially, is going back and forth between them so fast that it's an average.
That it's a delocalization.
Now, the way that we think about this in terms of our Lewis structures is by having one resonant form and another resonant form.
OK now, hold on.
So, here OK, let's do this, and this, and double bond on this side, good.
Chemists are very particular about how you use arrows.
And you have to be very careful in chemistry.
But here's a case where they really like the arrow.
And they really like curvy arrows in particular.
And what the curvy arrow shows you is what happened to the electrons.
So it's really just a representation of where the electrons went to get from one resonance structure to another, how they changed.
But it can be, actually, helpful to understand whether you really have a resonance structure and what's happened.
So for example, in this case, these are the two resonance structures for ozone.
OK so, what happened?
Well, to get from this structure to this structure, you can see, what's happened is that you can imagine this lone pair has gone onto that bond.
And this bond, where am I?
This lone pair has gone onto this bond.
OK, so I've made a double bond to get to there.
And I've made the double bond here to get to there.
Now what's missing?
Well, I've got to make the other lone pair appear here from this bond.
So you can look at your resonance structures and realize that that also happened.
And you can look over here, and I've got three lone pairs on that one.
So the only way to get that would have been, let's try draw these over here to make room, if that happened.
Those curvy arrows help us just see how the changes in the electrons happened in these resonance structures.
But the way to really think about this, and this is why I wanted to capitalize this word, is that the actual structure is a combination of these.
So let's see, I'll do this.
And the way you would write the actual structure would be like this.
OK, this, and this, and this, and dashed lines, oh.
And this is called the resonant hybrid structure, Resonant hybrid.
It's a mixture of the structures.
And it shows, with the dashed lines, the delocalization.
And if you want to be complete about this resonance structure concept, if you want to be complete, then you would also count formal charges.
And so you would see that the formal charge-- let me make sure I get this right.
So over here, we've got what?
6 minus 1, 2, 3, 4, 5, 6, 7.
So the formal charge here was minus 1.
The formal charge here, 6 minus 1, 2, 3, 4, 5.
So that's plus one in the middle.
And the formal charge here is 6 minus 1, 2, 3, 4, 5, 6.
So that's zero.
And over here, I'm not going to do the counting again, it's the same.
But the zeros and the minus ones have switched, as you can see.
Now, in the resonant hybrid structure, what's happened-- notice these are the two Lewis structures.
So you do have formal charge.
Formal charges are not zero everywhere, but that's OK.
This is the best you can do.
But you've got the two that are equivalent.
And they go back and forth.
And in this case, the way you would draw that is that you would have the plus is still there, but now you're sort of sharing the formal charge on the ends.
So if you want to think about this in terms of formal charge-- notice something about formal charge.
I did not add or take away charge, in this case.
I got it added up.
The addition of all formal charges, by the definition of formal charge, must be equal to the charge on the molecule, must be.
So if the molecule is neutral, then the formal charge must add up to zero, which it does, does, and it does.
Good way to check your work.
OK so, we can go further.
So that's the concept of resonance.
And I did it, as I like to do, kind of slowly.
And now I will give you another example.
But I'm not going to go through it as slowly.
And but oh, let's ask this question, so if I had-- OK so, here's two other cases.
Now, this one we're going to get through quickly.
Is this a resonance structure?
And I'm going to give you the answer, the answer is no.
And the reason is that resonance structures only involve the curvy arrow motion of electrons.
The curvy arrows showing us how electrons might change in this, but not of atoms.
Notice I cannot get from here to here without moving an atom.
That's not a resonance structure.
That is not a residence structure.
So we're done with that.
And we can put, well, let's put a little thing here.
Only change location of electrons, not atoms.
Let's keep this separate.
Not atoms.
But now, we get to another one, where we didn't change the location of atoms, but we did change the location of electrons.
And you can see the structure up there.
And I won't do this, because I just did it for ozone, but you can go through this yourself and show, with curvy arrows, what's happening between these structures.
These are three resonance structures.
These are three resonance structures And you can see how they go.
So you can see, like here, I had that extra lone pair on the oxygen, that might have a curvy arrow to here to give you the double bond there.
But as I did that, in order for carbon to keep its formal charge, to keep its formal charge, I had to do something with this double bond.
And so this is how you think about resonance structures.
And just to give you the final for that one, just show you what it would look like.
Once you've got the hybrid structure, it would look something like this.
So there, there, and oxygen with its two lone pairs, and oxygen with its two lone pairs, and the delocalized electrons.
And then you've got your charge.
And this would be minus 2/3, minus 2/3, minus 2/3.
Notice on these three oxygen atoms, notice that, again, the formal charge adds up to the charge on the molecule.
There it is.
Charge on the molecule, that's a 2 minus.
This is CO3 2 minus.
Formal charge adds up to the charge on the molecule, good.
OK, one more example, because now we're going to combine this and learn one other really important concept about Lewis structures.
Let's see if this, OK.
So here's an example-- do I have this up here?
No.
Here's an example, what if I had the thiocyanate ion?
This is a favorite.
So this is CNS minus.
Might as well write the name here, thiocyanate.
This is a favorite for demonstrating concepts about Lewis.
And you'll see why in a minute.
Because first I'm going to draw a Lewis structure.
This is a minus, so it's an ion.
First I'm going to draw a Lewis structure that seems to make sense.
But then as you'll see, there are resonant Lewis structures that also seem to make sense.
So I need to use some of the concepts we've learned to figure it out.
So if I draw this, let's see, I'm going to draw three.
So N, triple bond, C, sulfur, lone pairs, lone pair.
Or I could have N double bond C, double bond sulfur, lone pair, lone pair.
Or try to squeeze this in underneath, so that you can, put it here.
N, three lone pairs, single bond C, triple bond sulfur.
Now, these are resonance structures.
I can get them by drawing my curvy arrows and moving electrons around.
And so you might say, well, is this not just going to be an average.
Are these electrons delocalized according to their resonance structures.
But see, once we start thinking about formal charge, we get more insight.
So the formal charge here is minus 2.
5 minus 1, 2, 3, 4, 5, 6, 7 minus 2.
Here it's zero.
And here it's plus 1.
Sulfur 6 minus 1, 2, 3, 4, 5.
So that looks pretty bad, a large formal charge minus two.
OK over here, oh, I didn't draw the lone pairs, sorry about that.
Here's two lone pairs here.
We will not mess up the electron count again today.
And so here, the formal charge, let's see, this one would be minus one.
And in here, it's zero.
And over here, it's zero.
That looks better.
According to my rules on Wednesday, this looks like a more stable structure than that.
So even though it's resonant, I'm going to say that's probably not going to be part of the most stable ground state structure.
But let's look at this last one.
Zero, and over here, I've got zero, and over here, I've got minus one.
What do we do?
There's one last point that we need to learn.
And it has to do with the formal charges.
And it's a general rule in writing Lewis structures.
And that is that, let's see if I can fit it here, because it's very relevant to here, that atoms with the negative formal charge should be-- I'm not going to fit it here-- should be on the more electronegative ion, electroneg atom, not ion, atom.
So let's take a look and see what this means.
Let's understand it, first.
I just wrote something down.
Let's make sure we understand it.
So atoms with the negative formal charge should be on more electronegative atoms.
That kind of makes sense, right?
Because remember what our definition of electronegativity is.
Electronegativity is the desire of an atom to bring bonded pairs towards itself.
Remember, and it makes a molecule polar, polar covalent, for example, maybe ionic.
But anyway, it's that desire to bring bonded pairs towards itself.
So if an atom has a negative formal charge, then you think, well, that should be on the more electronegative atom.
Because the negative formal charge means it's got a little bit extra charge compared to when it was free.
And so if you look at this, oh, now you need to know electronegativities, something that we know.
The electronegativity of nitrogen, so chi, remember, of nitrogen is around 3.0.
And chi for sulfur is around 2.5.
And that tells us that this is the ground state structure.
All from the concepts we already know.
All from the concepts we already know.
Now, at the same time, the atoms that are more electropositive would want to have positive formal charges, in general.
So the same rule applies the other way.
OK now.
So that's the last part of Lewis structures that I want you to know about.
And it all ties together.
You can see resonance, this is why this is such a great example, you can think about resonance.
And could you move electrons around and get different structures?
Yes, but then those aren't actually equivalent, because they have very different formal charges.
And then two of them had kind of similarish formal charges, but one is more stable because of that.
Now why does this matter?
And I'm going to tell you an example of why this matters.
I'm talking about delocalization to stabilize.
And I'm talking about how the actual structure of a molecule isn't this rigid two bonds here, one bond there, that's not what that says, but it was in ozone.
But it's this shared equivalent bonds where the electron is shared and the whole system is lowered.
Now, there is one molecule where this is very much important and dictates all of its chemical behavior.
And that's benzene.
And back in the day, in the 1930s, oh, by the way, Pauling, electronegativity scale. we talked about him on Wednesday.
But back in the day, they didn't know what benzene was.
They could kind of measure some things.
But none of it worked.
And Pauling wrote a number of structural formulae have been proposed for benzene, but none of them is free from very serious objections.
The way they wrote stuff.
Look at these shapes for benzene.
If we don't know the right shape and why you have the right shape for benzene, then we cannot understand all of the mega amounts of organic chemistry that we do with benzene.
So this is what's happening with benzene.
You have these two structures that benzene actually averages over.
These are resonance structures.
Back in the day, they called them Kekulé structures because Kekulé, as you can see, was kind of close and thought about this as well.
These are resonance structures.
It totally dictates the chemistry of benzene, which then leads to massive amounts of things that we do with benzene.
Because with benzene, and this is just, we don't need to look at this in detail, this is an example of a few out of almost unlimited variations of benzene that we can make.
Because we can take one of those hydrogen atoms and put something on it, or two places.
And each time we do that, we get a different material, a different molecule that gives us different properties and different uses.
But all of this, even though we draw it, still with those lines, we know that inside, it's delocalized.
It's delocalized.
Well, it might change once you add stuff to it.
But by itself here, by itself here, it's delocalized.
And that sets up the properties of benzene.
Now OK, let's go a little farther.
So I like these last two here.
Naphthalene, now, you can keep on going, and keep adding benzenes, and we're going to get somewhere that I really love.
This is getting me excited.
Oh, look I'm going to add one there.
I'm just going to keep adding benzene.
Now I could stay here, oh, don't get benzene wrong.
I can keep going.
And if I kept going, I might get sheets of benzene that are now called graphene.
And if I stack those up, I've got graphite.
I've got graphite.
That's what graphite is.
It's these very large sheets of benzene.
Now, there are two very well-known phases of pure carbon.
One is diamond.
And the other is graphite.
Does anybody know which one is more stable?
How many people think graphite?
How many people think diamonds are forever?
I'm giving you guys some advice, especially like, you're out, and maybe you're out on a date.
And it's the weekend.
And there's a candle.
And you've talked about combustion.
And you've written that down.
And you talked about how long you have in that room, because you know about oxygen and the limiting reagent that it's either you or the candle.
We talked about that.
We also talked about how you need your periodic table on that date.
And how you may need your spectroscope, because they might give you an LED candle instead.
You need to talk about LEDs.
And I'm not saying that all of these conversations involving knowledge you gained in this class will lead to this place of seriousness, but it could lead to engagement.
It could.
And now, some people when they get engaged, one person buys the other a ring.
And sometimes that involves this material.
My point to you right now is when you go to the store, maybe it's Tiffany's, maybe it's somewhere else, and you buy that ring, ask for a warranty.
And make sure it's about 100,000 years, because after that time, that ring is going to turn into graphite, because graphite is the lowest most energetically stable form of carbon.
So I'm just, again, always trying to help.
But this is the most stable form of carbon.
And it's a bunch of benzene, but repeated.
Not benzene, the hydrogens are gone.
It's just pure graphene, but repeated in these beautiful rings.
And when we come back to hybridisation and molecular orbitals, which we'll start after exam one, you'll see other beautiful properties of structures like this.
By the way, speaking of seeing, you can see this material.
What are you throwing at this material to see it like that?
Not light, not photons, you're throwing electrons.
I just came across this the other day in Wired, New microscope shows the quantum world in crazy detail.
They took electrons and they shine it on these little particles of platinum and iron.
And they're able to literally see every single atom as they break apart the particle.
By just throwing electrons at it, they say, the transmission electron microscope was designed to break records.
Using its beam of electrons, scientists have glimpsed many types of viruses, et cetera, et cetera.
They got down to 0.4 angstrom resolution in that work.
It's a beautiful thing.
And being able to see these materials has revolutionized what we can do with them.
Diamonds and graphite makes me, when I talk about graphite, I get excited.
I get excited.
Now I'm working on my arm here.
So I got to go up that way.
And I got to go this way.
All right, hold on, hold on, let's go that way.
Now, wait a second.
I'm very excited.
Oh wait, there was a lot of noise.
I tried.
I got to work on my arm.
It's such a beautiful material.
Too excited.
We'll have more.
Now I don't mean to bring us down after why this matters and excitement.
But here's the thing, if there's one thing you know about chemistry by now, it's that chemistry lives in the fast lane.
And what I mean is, they know about the rules.
And they also know they're meant to be broken.
And Lewis and octets are no exception.
And so there are exceptions to Lewis's octet rule.
And I'll give you a couple of examples.
And it has to do with the concepts that we've been talking about, but in particular, like boron.
Boron trifluoride, well, that one, you could draw it.
If you wanted to, you could draw it like this.
And let's see, here, F, and you can do this, F.
But the problem with this is then, when you look at the formal charges, you've got a minus 1 there and a minus 1 there.
And so actually, what this structure does, is boron is like, you know what, I'm flexible.
I don't need my eight.
I can be OK with six.
As long as you keep sharing, fluorine atoms, as long as you keep sharing those electrons so we can make these three covalent bonds all equivalent, I'm OK.
And so this is actually-- and you can see, the formal charges on all the atoms here is zero.
This is actually the stable structure, even though boron does not, this does not satisfy the octet rule.
Chemistry, breaking the rules.
So boron is said to be happy.
Boron can be, what is called in this world, electron deficient.
And by the same token, you can go the other way.
And sulfur likes to go the other way, because sometimes sulfur, so there we have sulfur tetrafluoride.
So I'm using the same external atom, but sulfur also prefers to look like this and to have one extra lone pair there.
And now we can go like this, and like that, and like that.
And so what sulfur will do, oh boy, lots of dots, and so what sulfur will do is it will form what's called an expanded octet, expanded octet.
And this will become also, a little bit-- we'll sort of understand this also a little bit more once we look at shapes.
Shapes are going to play an important role in molecular stability.
But for now, I just wanted you to know that there are exceptions to the rules.
And in particular, as you go beyond the first couple of rows, so you go down in the period table, you do find atoms more willing, like sulfur, to have an expanded octet in order to reach the lowest energy state.
Now, in the last two minutes, I come back to this.
Let's just bring it all back home around Lewis, and around electronegativity, and around all these covalent bonding concepts.
And what I want to leave you with is what was kind of the point, part of the point of your goody bag.
Which is, I wanted you to get your hands on these materials so you could touch and feel the differences and see that actually it's the properties at the end that are also very important for determining what a material is.
So you can make rules like electronegativity difference less than 1.6 is going to be polar covalent or non-polar covalent, or greater than two, it's probably ionic.
But at the end of the day, you've got to come back to properties.
I'll put up a slide showing this, but ionic solids are hard, brittle, and solid at room temperature.
Covalent solids can be gases, liquids, or solids.
Covalent solids have a low melting point.
Ionic solids are typically high.
Covalent solids are poor conductors.
Ionic ones are usually insulators as a solid.
This is what you need to also do.
You can't just go by one metric.
You got to look at the properties.
It's what Mendeleev did.
And it's what chemists have always done to make progress.
OK so, on that note, have a great weekend.
And see you at the exam.
[CROWD NOISE]