Flash and JavaScript are required for this feature.
Download the video from Internet Archive.
Description: This lecture covers x-rays which have the right range of wavelengths to image at the atomic scale.
Instructor: Jeffrey C. Grossman
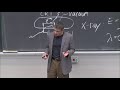
Lecture 20: X-ray Emission ...
How's everyone doing?
[APPLAUSE]
Parents only, how are you doing?
[APPLAUSE]
How is it that the parents were louder than the kids?
Kids, how are you guys doing?
[APPLAUSE]
All right.
Welcome, parents.
Welcome, parents.
I'm really excited to have you here in class.
This is 3091, Introduction to Solid State Chemistry.
And today we're going to have a little bit of fun.
As you can see, we got some activities.
Now, the way I like to frame this-- are there any other seats?
No, not so much.
But make yourself comfortable.
The way I like to frame this is you've got a lot of activities going on already.
This is the parent weekend.
So if we look at-- why is that happening?
If we look at the-- oh, that's not good.
OK, there we go.
If we look at the MIT family weekend, there is activities.
And so what I thought is that what we could do in this class-- we've got to learn a little bit.
We're going to learn about x-rays today.
But what I thought we could do is also add some ideas for activities that you all could do with your kids.
But it doesn't even matter.
It could be with any kids.
But just more activities to do to give you more options.
And so in particular, I've got five suggestions for you that we'll talk about today.
The first one is staying warm.
It's gotten really cold, so we're going to talk about staying warm.
And then the next thing we'll do is we'll appreciate the fall and all the colors.
And we'll cook.
Cooking is such a wonderful thing to do.
I hope you guys can do it together.
We'll talk about that.
And we'll talk about phones and how they crack their screens.
And we have some sort of larger versions here that we'll talk about.
And of course, it wouldn't be complete if we didn't blow bubbles, because that's a really fun activity no matter what age you are.
So that's our plan.
That's our plan today.
Now let's get started.
So let's start with the first activity, and it's staying warm.
So I love candles, and I think a lot of us have seen candles.
The difference is between everyone else's and your kids who are in this class who see candles is they don't see candles.
They see C25 H52 combusting.
And your kids now, when they see a candle-- if you guys go out to dinner and there's a candle on the table, the first thing-- I guarantee you the first thing they're going to do is assess the volume of the room, figure out how much oxygen in grams there is in that room, and they're going to decide which one is the limiting reagent, the candle or you.
And they'll make a choice.
They may get you out of the room because you don't know.
You've got to know that.
And of course, they'll have their periodic tables with them at the dinner.
Don't be surprised when they take it out.
Now, your students-- oh, yeah, that's perfect.
Your students also know-- your kids, my students, your kids-- also know that a lot of the earliest chemistry, going all the way back to the times of Democritus thousands of years ago, the way it started was because people were breaking things and smashing things and lighting stuff on fire.
And we've got the whole periodic table in this class, but we still know that the most fun element of all to light on fire is the very first.
It's hydrogen.
And so I thought I would show you what that's like.
OK.
So here's hydrogen.
And like I said, this is also-- it's a way to think about-- oh, that's a beautiful thing.
Is that a beautiful thing?
I've got to do it again.
And as your kids know, I can do this a lot.
But it's just-- and my hand doesn't-- I don't feel it because of the heat capacity.
But that's another class.
But what we're interested in is combustion.
So there's that one there, too.
So this is all about the context of staying warm, of course.
You don't need to light hydrogen on fire to stay warm.
But I also have suggested discussion topics.
Because I don't want you just to do these activities.
I want you to be engaged.
It might have been a while since you guys have connected-- I don't know-- in person.
And so here, maybe you don't know what to say at first.
And so for example, you could talk about balancing chemical reactions.
You could talk about the concept of a mole.
And you could also talk about limiting reagents, which are really, really important in life.
So that's my first suggested activity.
Like I said, we'll have five new activities.
That's the first one.
But like I also said, we've got to learn something today.
And students, this is not on the exam, as a reminder.
But we do need to learn what X-rays are.
Because next week, we're going to be shining them on stuff.
So today, we're going to learn about how X-rays are generated.
And it all starts with the very first selfie.
This is it.
Does anybody know who that is?
That is Anna Rontgen. That is the wife of Wilhelm Ronten.
That's the very first X-ray ever taken.
That's the very first X-ray ever taken.
And Wilhelm Rontgen was playing around with cathode ray tubes.
And so he-- I love this quote of him.
He said, I did not think.
I investigated.
And that's-- now, we think.
Of course we think.
But what we do in this class is we investigate.
We're explorers.
Were explorers of chemistry and how chemistry connects to what we see in life and what we're doing every day.
And so how did Rontgen do this?
OK.
Well, literally, it was a dark and stormy night, literally.
And he was in the basement in his lab.
That's him.
And it's going to be stormy tomorrow, so you guys could even try to think about how it felt. And so what he did is he-- everybody was playing with these cathode ray tubes.
We learned a lot about cathode ray tubes.
So here, you put some kind of cathode here.
And then there is an anode here, and you hook it up to a voltage.
And what happens is electrons fly.
This is a cathode ray tube, so it's the CRT.
Electrons will fly off of the cathode and go here.
Now, if they go through the anode, and then you put a screen here, those are the very first cathode ray tube experiments.
And we talked about how this led to the discovery of the electron itself.
But what Rontgen did is he said, now, hold on.
What if I changed-- I put a voltage here.
What if I amp that up?
What if I just keep going?
Oh, by the way, there is a vacuum inside of here.
There has to be a vacuum.
And if I am the voltage up-- you know what?
Actually, I'm going to put a metal here for the anode.
I'm going to make that some kind of metal, and I'm going to hit the metal with the electrons.
Now, this is exactly what happened.
What happened is the room glowed.
The room glowed.
So Rontgen had-- showing you there, the lights are off.
The lights are off, but the room was lit.
And he said, oh my goodness.
What is going on here?
What is going on?
And what he decided-- he literally discovered a new type of ray, a new type of light ray.
And because he didn't know what to call it-- he didn't have a name on that dark and stormy night-- he wrote down the letter x, which he regretted later.
He really did.
Because everybody started calling it-- he didn't know what to call it, so he called it an X-ray, which is what happens when you do this experiment with a very high voltage.
You get rays that came off of this and illuminated the room.
And he said, I don't know what to call it.
I'll put the letter x for now.
And that stuck.
Now, these X-rays are unique.
They're unique because they have very high energy.
They have very high energy.
So the X-ray energies-- the energies are in the range of 100 eV to 100 KeV.
That's a big energy.
But we know how to go back and forth between energy and wavelengths.
So we know that that means that the wavelength range is between 0.01 and 10 nanometers.
And that's what's really important.
You came up with a source that has wavelengths in this very, very special regime.
This is a very special regime because it's the distance between atoms.
Now, there's two types of x-rays.
But first, let's-- at the time, you might think, people-- why wasn't he wearing anything, like at the dentist?
Why wasn't he wearing anything?
They didn't know that radiation could be dangerous.
And so if you look at the time, there were ads like this for a system to remove the hair.
And it was advertised as effective, safe, and painless.
This is-- those are X-rays, one of the first uses of X-rays.
This is a token to get into a bath that's called a radium bath.
It's a water bath where you have radium that you either drink or is in the water itself.
And look at what was happening in Paris.
Radium cure, a fad of Paris society.
Patients play bridge and take tea for two hours daily-- daily-- in a drawing room.
This is what it says.
December 9th.
The afternoon radium cue is the latest craze in Paris society.
The popularity of the treatment, new to Paris, has devolved quite suddenly and is due, no doubt, to the fact that it is exceedingly pleasant.
Oh, France.
Right?
They didn't know.
They didn't know.
And it was the same with these X-rays.
What is this thing?
Well, it turned out it was a very, very important type of light.
And because of its importance, Ronten won the first ever Nobel Prize given in physics.
There is an element, number 111, named Roentgenium.
And 60 years later, the DNA was discovered-- the structure of the DNA, the double helix was discovered because of X-rays.
So the impact of X-rays is tremendous.
Now, the next activity, as I mentioned, has to do with enjoying the fall.
Now, the thing is that New England has beautiful colors.
And so if you haven't taken a stroll around the parks, or maybe you go up to a nice hike-- you'll see these beautiful colors changing in the leaves.
But the thing is that in our class, your kids, they don't see that anymore.
No.
They don't.
Because when they see colors, they think about electron levels.
That's what they think about.
They can't help it.
They cannot help it.
So when they see orange or red, all they're thinking about is, oh, my goodness, there is an electron level here, and an electron getting excited, and it's coming back down and emitting a photon, and how those levels are changing as you go from one leaf to another, and how chemistry is at the heart of it all.
Chemistry is at the heart of it all.
And so that's what they're thinking of.
And we can use chemistry.
I don't know if we have the next one ready here.
We can use chemistry not only to change the levels of electrons that are in this material, which then changes the color of light that comes off of it-- it's all about the electrons-- but we can use chemistry to control how quickly that happens.
Nature does it on this yearly time scale.
We can do it on any time scale because of chemical kinetics.
So we're going to get that experiment out here in a second.
Meanwhile-- oh, there it is.
Can we give the helpers a huge hand?
Because they are-- thank you, guys.
[APPLAUSE]
All right.
Now what do I do?
And that's it?
This is pre-mixed?
Oh.
OK.
Now, this is an example of how we can change-- we can control the color of a material using chemistry.
We can control when-- do I pour it all?
Do I pour it all?
OK.
Why not?
Yeah.
OK.
Now I want you to focus on the larger bottle.
Nothing is happening.
Keep watching it.
You've got to keep watching it.
See?
That's an example.
But your students, your kids are seeing this and they're saying, no, it's all about electron levels.
I've got to go and write down those electron levels.
I don't care if it's a Bohr model or a semiconductor bandgap.
I've got to figure out how that happened.
How did the color change?
I changed the electron levels in the liquid.
That's what I did.
And I controlled the kinetics.
So my suggested topics for this one of discussion-- chemical kinetics.
Now, we're going to talk about chemical kinetics later in the fall, but you guys can get a head start this weekend.
Because that's what happened there.
We controlled when it-- it didn't happen right away.
It happened later.
Why?
Acids and bases, also coming later, get a head start.
And then how to paint with electrons, because that is what we're doing.
We're changing how electrons interact with the material to change the color.
We are painting.
And that's what nature is doing with its leaves.
Now, you might be on this walk, and you might be strolling around, and you might not go into the countryside.
You might just be down Mass Ave. Now, if you're walking down Mass Ave from here, and you go not towards Boston but the other way, you get to another school.
Now, I've got to-- I have talked to your kids about this already.
What I want to do right now is address the parents.
You have activities you're going to, right?
And some of them may involve-- I don't know, a dinner or a party, or a lot of other parents.
Some of those parents may have kids that go to the other school.
Now, here's what I want to tell you.
It might not be their fault. It might not be their fault.
I'm just saying we do the best we can as parents.
We do.
We do everything we can.
We did everything right.
And then you realize sometimes, kids have to make their own mistakes.
So I just-- and the last thing I'll say about this-- look, be nice, because they're probably going through a really hard time.
So that's what I want to say about the stroll.
You might wind up down there.
I don't know.
OK, back to our X-rays.
Where were we?
So x-rays are coming off of this metal.
It's super high voltage.
It's a a dark and stormy night.
What happened next is that we had to figure out what were the properties of this light.
And what we really mean are things like intensity and wavelength.
Now, I wrote down ranges there.
But basically, what you have with X-rays are two types.
You have type 1, which is called continuous.
And it arises continuous-- continuous.
And it actually-- this is a pretty good name, because it's a continuous energy up to a point.
It's a continuous spectrum.
So what do I mean by that?
Well, what I mean by that-- to understand that, we have to know how we get it.
There are two types of X-rays.
In this first type, what we have is we have some atoms with a bunch of protons in there.
This is like that metal atom.
And I've shot really high-energy electrons at it.
But that atom has electrons sitting around it.
We know that.
1, 2, 3, 4, 5, 6, 7, 8.
OK, and then again.
And these are the quantum levels.
This would be n equals 3, n equals 2.
And in here, we've got n equals 1.
But now, here's the thing.
I'm shooting high-energy electrons at this atom.
So one of the first things that can happen is nothing at all.
So if I shoot it, say, up here, way away-- if it's all the way up here going way away from the atom and doesn't feel it, then it might just come through.
It might have some energy-- call it e0-- and it just came all the way through.
It didn't interact.
Well, that's kind of boring.
What if I interacted-- what if I'm out here, and I interact-- I got through some of those outer electrons.
Immediately, what do I see?
If I'm a negative charge, I see this positive charge, and I'm like, ah, that looks good.
It's an attraction.
And so the electron curves like that.
Now, that means that it accelerated.
This is not a electron stuck in an orbit.
No.
This one was free, and it remained free.
But because it got inside of the electron cloud of the atom, it saw a a positive charge.
And that bends it, and that accelerates it.
And when something that's charged accelerates, it gives off a photon.
It gives off a photon.
So that might be some kind of energy that it has left over that we call e1.
And this would be like the photon 1.
And we know that it's lost energy to the photon.
It's decelerated.
It's decelerated.
So we know that e0 is greater than e1.
But I could have shot another one through, and this one got even luckier.
These are my electrons, incoming electrons.
This one got even closer to the positive charge, felt an even stronger attraction, and decelerated even more.
And so it also emits a photon.
And so we know that e2 must be smaller than e1.
It's left with less energy.
Now, what you're doing is you're decelerating electrons by having them pass through the cloud of charge of an atom.
And that deceleration emits photons.
And you can see right away what I'm doing is I'm breaking the electrons.
Well, that's exactly what this kind of radiation is called.
This radiation is, in fact, called Bremsstrahlung.
And that literally means braking radiation.
Braking radiation.
It means breaking.
Well, if I take this very simple picture, and I try to make a plot-- like I said, if I want to understand my light, I've got to know about how it behaves as a function of, say, wavelength or frequency.
And so if you plotted the wavelength with the intensity, well, you can see right here I'm going to get-- OK, so I'm going to get some photon that comes out that has some lambda, and then another photon that comes out that has another lambda.
But you can see that that electron could have been braked at any loss of energy.
And so what you get is something that is very continuous up to a point.
This is the shape that it looks like.
So I can get-- this is lambda.
So if lambda is out there, this would be like lower energy photons.
And up here, it's higher, higher-energy photons.
Now, these are all x-rays.
They're in this very high-energy range.
But you've got this continuous spectrum.
Because the electron could have been slowed down in any way except higher than this point.
Why?
Well, that's just determined by how much energy that initial electron got.
Because you can imagine this point is a special point.
This represents a fully stopped electron, fully braked, fully stopped electron.
So that incoming electron has transferred all of its energy to the photon.
And because this is higher energy, that's as high as it gets.
That makes sense, right?
OK.
We have a name for that too.
That's the Duane Hunt limit.
And basically, it says that the energy of-- the maximum energy, e max of the photon that can come out is equal to the electron times the voltage, the charge of the electron times the voltage across, ev across the tube, and that that is equal to hc over lambda min.
This is just saying max energy equals hc over min wavelength, energy wavelength.
So that's one type.
And if I had-- but now you can see if I shot it, now I'm bumping up the voltage even more, and I'm shooting it with higher-energy electrons.
Well, you can imagine that if the electrons I shoot in have a higher energy, then that point is going to go in and in like that.
So this would be Bremsstrahlung radiation, and this would be increasing incident-- inc?
That's not good.
Incident electron energy.
And I will put a box around this so that it's increasing, that arrow going like that.
So if I shoot it with higher and higher-energy electrons, I'm still going to have a minimum lambda, but it's going to get smaller and smaller.
Continuous x-rays.
OK.
That's type 1.
Now, speaking of breaking-- now, speaking of breaking-- OK.
Now, here is my third activity, and it's cooking.
And I love cooking, and I hope you do too.
It's a really fun thing to do.
And I think that I love cooking with cast iron.
So maybe you've got a piece of cast iron in your dorm that you can pull out, and pull out a recipe, and start making something.
But what I want you to think about-- and this is the thing-- is your kids, our students don't see it as cast iron anymore.
They see it as iron with interstitially doped carbon.
That's what they see it as.
And so I want you to see it that way too.
And then that gets you thinking.
How is this cast iron so strong?
And oh, wait, the early chemists-- I heard they smashed and they lit things on fire.
How could we break this cast iron?
Could it break?
Could it break?
Should we be worried about it?
And so what I think you should do-- oh, yeah, there it is.
Let's take this.
You take the-- I don't have a pan, but I have this.
Is this filled?
OK.
And you take out a hammer because you have a hammer in the closet.
And you go like this.
And nothing happens.
And then you do it again.
You say, well, you know what?
Maybe we need to go a little bigger.
And you go into your closet, and you find a sledgehammer.
And you say, you know what?
I think if we just use this handy sledgehammer-- hopefully not in your dorm-- then maybe we'll break it.
And so you keep hitting-- not over the electrical.
And you hit it again.
And I won't use-- I won't go extreme here, but I will tell you that I've done it before.
And nothing happens.
Nothing happens.
There's not even a scratch on it.
But these are your kids who go to MIT, not another school.
And they know-- and they know that intermolecular forces have power.
They've got a lot of power.
And they know about bonding.
And they know about the hydrogen bond, and the power of the hydrogen bond, and how strong it is, and why it is.
And then they start thinking about water.
I wonder if we could leverage the power of the hydrogen bond.
Now, do we have liquid nitrogen?
We do.
OK.
So they put it together that we might be able to leverage that power of the hydrogen. They knew.
They saw the gecko.
They say, the London dispersion lets a gecko climb a wall.
Well, then hydrogen bonds might be what it takes to break the cast iron.
All that you need is to pull the liquid nitrogen out of your dorm closet, and-- yeah, OK.
You want to go ahead?
Yeah.
And fill it with water because you need to take advantage of the power of the hydrogen bond.
And then let it sit, of course with the blast shield that you also have handy.
OK.
Well, let's see how this goes.
And we might take a-- we'll see what happens.
Again, this is a bonding moment.
This is a hydrogen bonding moment.
But this is where the two of you-- the three of you, the four of you-- it could be a huge group.
This is where you talk about things like the crystal structure of iron.
This is where you talk about things like intermolecular forces.
And we've learned them all.
We learned about them all in this class.
Where you talk about the power bonds and the power of the bond between you as a family.
This is it.
Just do it on this side of the blast shield.
OK.
Now we'll let that sit.
We'll see how that goes.
And OK, yeah.
That's good.
Now, where were we?
Oh, right.
Oh, there's another type of X-ray.
There's another type of X-ray.
And the other type of X-ray-- that's the only board left.
The other type of X-ray is from a different phenomenon that also has to do with-- it also has to do with the atom and the electron.
Because the one type is because the electrons are coming through this cloud, and they get a little attracted, and they lose energy, and they give that off to an X-ray photon.
But see, the other kind comes from a direct excitation, the same kind that we learned about when we looked at the Bohr atom.
And so this is drawn here.
Now, there's a very important thing that happens.
As we've learned in this class, when you go from one area of expertise to another-- like crystallographers don't like commas.
They don't like commas.
Don't upset a crystallographer.
It makes no sense.
It's not worth it.
This is the same thing with X-ray specialists.
Don't use n equals 1, n equals 2, n equals 3.
Use KLM, because they prefer that.
So the KLMs there are simply the quantum numbers, the principle quantum numbers.
So 1 is k, 2 is l, and 3 is m.
And all we're doing is the same kind of excitations as drawn there very well.
So you see the incident electron comes in, and now it's got enough energy to excite one of those core electrons.
It's got enough energy to excite it.
And so what it does is it trades energy with that core electron.
The core electron goes up, and then it comes back down.
Now, if we had, say, an m level-- so this is n equals 1, 2, 3.
So if we had-- now I can draw it.
If I had some electron in my k shell, my n equals 1 shell, and that electron got bumped up to an l shell, well, now you know-- from our work with the Bohr atom, you know that when this goes back down, it's going to emit a photon.
But this is very different now.
This is very different.
This photon has only that energy, nothing in between.
It's not continuous.
I'm getting used to wearing this.
It feels good.
It feels pretty good.
So I could have had an electron-- instead of going to the l level, maybe it went all the way up to the m level.
And then from there, it cascades back down, and it emits a photon.
And the way this works in terms of labeling is because it goes down to the k level, these are k photons.
And we go in order.
So this is the least energy k photon.
It ended in k.
So that's a k alpha or k-- I'll just write it out to be clear-- k beta.
Oh, I hear sizzling.
Oh, that's because you're pouring.
That's good.
More liquid nitrogen is never a bad thing.
That's great.
No, no, I'm good.
You guys are doing such a good-- now, OK.
So if it's k beta-- because it went to k, it ended at k.
It gave off a photon.
The photon is either alpha, beta, gamma, depending on which one.
But now here's the thing.
You know if you go back to that plot of energy-- of lambda versus intensity, well, you know that there's nothing in between.
There are no electronic states in here.
So you can't excite in between.
This is forbidden.
It's forbidden.
So unlike the braking kind of radiation, in this case, it's very, very discrete.
And so now, if we plot what you get from this, well, you're going to get a k alpha peak that's very, very discrete, and you're going to get a k beta peak that's only at the one energy that is the difference between these levels, k alpha k beta.
So that's another kind.
This is a type 2.
This is second type of X-ray.
OK.
It sounded interesting.
We'll let it keep going.
We'll let it keep going.
It's good because it gives you a time.
And I hope-- you guys can just leave it.
I hope that during this time that you're here doing these activities, don't have your phones.
Put them down.
Listen to the sounds of cast iron popping.
You can't appreciate it if you're on your phone tweeting it or DMing it.
You can't do that.
It's not going to happen.
It won't be as much of a bond.
Did I get it right?
No?
OK.
So we got our second type of X-ray, and it's discreet.
And we're going to put them together in a minute.
But first, we've got to talk about glass.
Now-- oh, more.
OK.
Good.
Good.
Keep going.
Oh, glass.
That's perfect.
The thing is that-- well, a lot of times this happens to your phone.
And we know about crystals in this glass.
And this is the one-- if you were willing to pay hundreds of dollars more for your iWatch, you can get a quartz screen.
This is quartz.
This is what we know now.
We don't look at the Apple ad.
We look at this in this class.
I'm good.
We're good.
Yeah, thank you.
And so we can hand this out because we like to touch and feel the chemistry in this class.
That is quartz.
That is quartz.
That's not glass, by the way.
And that's another thing we're going to learn.
Glass means amorphous.
It means not crystalline.
It means not crystalline.
That's the thing on the right.
Now, quartz has a lot of really great properties.
It's a very strong material.
But it's a lot more expensive, which is why this happens.
And you've got all this glass, this amorphous SIO2 that is-- oh, that was a good one.
Yeah, I see water coming out.
But there are ways to try to make that stronger, to try to make the amorphous SIO2 stronger.
And there's no better way to think about-- and I don't want you to break your phone.
But if you think-- speaking of phones-- if you think about glass, you're probably thinking about baseball.
And if you're thinking about baseball, you're thinking about the Red Sox, of course.
Right?
Am I right?
And so I brought glass, and I want to think about how glass breaks.
That's what we're going to study in a couple of weeks in this class.
But I thought let's bring this to life.
But I need some volunteers, and I'd like some parents to come down.
I need some baseball arms, some people who can throw this at the glass.
It's OK.
Come on.
I need some volunteers.
Come on down.
There you go.
You don't look quite like a parent, but it's OK.
All right.
OK.
Now, OK.
We need to get-- let's get you a little further back.
And we're going to have you put this on.
This is for aim.
And what I want you to try to do is throw this as hard as you can at that piece of glass on the left.
Can you do that?
All right.
Really let it go.
Now, hold on.
Hold on.
Let's move you right over here.
The one on the left right there as hard as you can.
OK, that's all right.
Try it again.
We get multiple tries here.
We'll take turns.
We'll take turns.
OK.
Try again.
OK.
That was good.
I think we need to try a little harder.
One more time, and then we'll get-- what parent?
That's the annealed one, isn't it?
Last year it didn't break, either.
Thank you very much.
Who's next?
I need a volunteer.
Come on down.
Oh, you look too happy not to try.
You look too happy not to try.
Can you hand those to this gentleman right here?
All right.
Come on down.
Now, we're going after that piece of glass on the left.
And we're going to see if it's a good piece of glass for your phone.
OK?
All right.
Let's let it rip.
Oh, yeah.
That's all right.
Try again.
Try again.
Multiple tries.
You've got this.
Oh.
That's very strong glass.
Try one more time.
Really?
OK.
Let's try the one on the right there.
Let's see if that one breaks.
We might have them reversed.
We don't have them reversed?
No?
All right.
Let's try that one.
OK.
All right.
Thank you very much.
[APPLAUSE]
Now, does anyone else want to try?
Apparently we have very unbreakable glass.
Maybe we've invented something new here at MIT.
The sledgehammer?
Oh, that's a great idea.
No.
But there's a reason for it.
Because, see, what we're going to learn is that baseballs aren't the only way-- even though this is Boston, the Red Sox-- it's not the only way to break glass.
Now, I don't know what's going on with this one.
This is tempered.
That means that it's been treated.
It's been treated so that it's got super strong mechanical strength this way.
You saw that it didn't break.
Of course, neither did that.
But when a glass is tempered, as we'll realize, it has to do with putting certain chemistry inside the glass, ions, that are bigger, that causes a strain in the material, that tighten the surface.
And so there's all this stress built up, and it's all at the very edge.
So this part is super, super strong.
But this part, just a simple little tap-- I didn't even-- I barely even touched it.
And so that is-- now, so the moral of that story is glass might be stronger than you think, I guess, in this one.
And also, don't tap the edges of glass, especially if it's tempered.
Because that's what makes it so tough on this on the surface.
But the strain builds up.
And if you relieve it with just a tiny, little tap, boom.
Now, where were we?
Discussion topics-- crystal versus glass, ordered versus disordered, substitutional dopants.
That's how to temper glass.
That's what's in your iPhone glass.
And of course, the Red Sox, which are on tonight.
And you should watch game three.
Now, the thing about these peaks is that these are called characteristic.
And what's nice about them is, in fact, that no matter what, they only depend-- I don't care how much energy I send in.
These peaks only depend on the metal atom.
So Ronten put in a metal, and he'd always get the same peaks if I give it enough energy.
Maybe I don't have enough energy to excite anything from down here.
Then you don't get these peaks.
You still get Bremsstrahlung, but you don't get the characteristic peaks.
They're called characteristic because they're characteristic of the metal.
Now they're dependent on the chemistry.
If I put in different metal in, I'm going to get different Peaks And so with these two types of X-rays, I've got a new kind of light that I can shine on materials.
I've got a new kind of light that I can shine.
And the light-- when I plot it all together, it might look something like this where maybe these are the peaks-- uh oh.
OK.
Maybe these are the peaks due to going down to K, and these are the ones due to going down to L.
So those are K, and these are L. and the rest is continuous, and they're on top of each other.
And I get it all.
But with that new kind of light, I can do new things.
And that's very important.
And that's what we're going to do after the exam, which is on Monday.
The exam.
Don't forget about the exam.
You're doing all these activities.
You've got to think about the exam.
Why is this important?
This is important because we will be shining x-rays as a source of light onto crystals.
And there's a very important thing that happens when you shine light onto something.
And you can see it here.
Light is a wave.
Light is a particle.
Light is a wave.
Light is a particle.
Have that discussion too.
But here we're looking at it as a wave, and waves diffract.
That means that they can add or cancel each other, right like water waves.
But it depends on the size of the thing they're interacting with.
If the size of the thing they're interacting with is the same as the wavelength of light, then you can get a diffraction pattern.
And so when we shine X-rays, which have wavelengths that are the same as the spacing between planes in a crystal, well, then it interacts.
And it can diffract.
And that allows us to determine the structure of the crystal.
That's called X-ray diffraction.
And this is how it all started.
It started with the Bragg father and son pair, who actually said in their paper the crystal is, in fact, acting as a diffraction grating.
And from that, you can literally tell us which kind of crystal it is.
I want to point something out here.
That's a father and son.
They both won the Nobel Prize together for this discovery.
Now, I'm not putting pressure on you guys.
I'm just saying it's not all about the kids.
The parents got to step up too.
So that's Bragg defraction.
And that is one of the most-- X-ray diffraction-- what we're going to do with these powerful sources of light, this is one of the most important ways to characterize crystals that we have.
And just as a reminder, I mentioned the exam.
Don't forget-- this is what we're talking about.
These planes-- notice the negative.
Remember the 1, 1 bar?
Don't forget about negative directions.
These are the things that we're diffracting off of, literally the crystal planes.
So just a reminder not to forget about that.
But I can't end by talking about exam one, so I have to end with something happy.
And bubbles always make us happy, always.
I love blowing bubbles.
I always will.
I always have.
But we don't just blow bubbles in this class.
We don't just blow bubbles.
We really blow bubbles.
And so what we're going to do is we're going to show you how we like to blow bubbles.
You want to do this?
You go ahead.
Now, what we like to do is we like to really blow bubbles in this class.
And so we're going to use exothermic reactions to blow bubbles.
And I think that's really a good way-- you go ahead.
You go ahead.
You're the one.
All right.
That's the only way to blow bubbles.
That's combining an exothermic reaction with bubbles.
That's happiness right there in a bottle.
That is happiness.
That is happiness.
[APPLAUSE]
Oh, yes, and look at this-- success.
Success.
The power of the hydrogen bond.
OK.
I hope you guys have a fantastic weekend, and I hope you enjoy yourselves and follow some of these discussions.
[APPLAUSE]