Flash and JavaScript are required for this feature.
Download the video from Internet Archive.
Description: This lecture explores how similar bonds dissolve similar bonds.
Instructor: Jeffrey C. Grossman
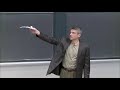
Lecture 28: Introduction to...
Today, we are going to continue thinking about reactions.
Just to remind you, what did we do on Friday?
So we introduced-- here's what we did last time.
We talked about constant-- we talked about reactions.
And there, on Friday, we were really interested in rates-- how fast is it happening.
So we talked about things that affect the reaction rate-- concentration.
Concentration versus time, and how you can measure that.
And you can have rate loss based on experimental measurements.
And that can tell you the reaction order.
We talked about zero, first, second-order reactions, the rate constant, and then we also talked about the temperature dependence.
And at the very end, the role of a catalyst in lowering that activation barrier.
Today, we're going to talk about how things dissolve.
So we're going to talk about solubility, and this has to do with reactions because something dissolving is a reaction.
But what's going to happen is we're going to talk about how that reaction finds its happy place.
And we know a happy place is a good thing, and it's called equilibrium.
And so that's going to involve talking about things like equilibrium constants, dissolution, we're going to look at some examples with ionic compounds-- so salts.
We're going to be dissolving salts in water and looking at how to think about how much of that dissolves in terms of the equilibrium constant for the reaction.
So that's the goal today.
I want to start, though, by talking about why this matters, and that's directly related to your goodie bag.
So this is something some of you may have seen.
The data here in from the '50s is from the Mauna Loa measurements, the top of a volcano.
And this is CO2 concentration, parts per million in the atmosphere.
But if you dig into the ice-- we're going to be doing a lot of that here in Boston over the next three months.
But if you dig deeper into the ice, in the cores, you go back in time, and it's a beautiful thing.
The ice as you go down is ancient atmosphere.
That's cool-- because there's little bubbles.
Remember, we talked about the half life of carbon-14 that's trapped from a living thing breathing it in.
Well, you can trap directly the little bubbles of atmosphere in the ice, and that's what happens.
And that's why we know what CO2 levels-- we're going back almost a million years here.
It's 800,000 years of data.
And this is a graph that I think many of you have seen.
It's going up, a lot.
And here it is.
Now, this is just zooming in on the last 50 or so-- this is just the data, the Mauna Loa data.
And The reason is because here we also have another kind of data.
So there's a lot of attention paid on CO2, and there should be.
There is a lot less attention paid to what that is doing to our oceans.
And this is a reaction that we will talk about more when we talk about acidification.
So when we talk about acids and bases, which is coming up later-- later being Wednesday and next week-- we'll talk more about this.
But I just want to tell you that when you have CO2 in the atmosphere going up, then you also have CO2 in the ocean going up.
So the CO2 in the ocean-- the CO2 in the atmosphere is getting absorbed by the ocean.
So what?
No, it's a lot of what, because what happens is the CO2 in the ocean-- the more CO2 in the ocean-- is making the ocean more acidic.
So if you just write this number down-- because it's so astonishing.
Past 200 years, the ocean has become 30% more acidic.
Now that is the fastest-known change in the ocean chemistry in 50 million years.
That's a big and very sudden change.
So the ocean is absorbing about 22 million tons per day of CO2.
And that number is going up.
Why does that matter?
Maybe it will do to the oceans what it's doing to the atmosphere and making things a little warmer.
No, it's doing more, because when it acidifies, then it has a direct impact on things like this, which is a pteropod.
You look at the food chain of the ocean-- there are three things at the bottom.
That's one of them.
I mean, there's plants.
There's photosynthesis.
And then there's the bottom of the food chain.
Now you know what's going to happen when the bottom of the food chain disappears.
This is one of the main kinds of animals that lives at the bottom of ocean because they've got these very small calcium carbonate shells.
If you make the ocean just a little more acidic, like we're doing, those shells don't survive, and those animals don't survive.
So that's what happens to a pteropod.
At the rate we're going-- like decades of time from now-- that's what happens.
So what you have in your goodie bag is a way to see this a little bit more accelerated.
So here's your goodie bag.
So I wanted you to-- I wanted you to see what's happening in the ocean, and you have now the tools to do that.
So in your goodie bag, you've got citric powder.
Imagine you take a lot of limes, and you squeeze them, and then you dry it all out.
And that's what you got-- citric acid.
Now you're going to mix that with water to change the ph.
And you're going to dissolve this powder.
That's the topic of today's lecture-- dissolution and equilibrium.
So you're going to mix that into water, and you've got a scale from a previous goodie bag.
So my hope is that you've been using that on a daily basis.
You take it with you places, and you can use that again here.
And you'll need that, because I want you to see how these shells dissolve.
And if you lower the acidity more-- we don't want to wait 40-- well, you can wait 45 days, but I want you to be able to do these experiments in minutes.
So we're going to go a little lower in acidity, in ph, but you're going to get the same result.
You're going to dissolve these shells.
Some of you are going on planes, and there's white powder and a scale involved in this goodie bag.
Please don't put that in your carry-on.
It's just not a good-- it really isn't a good idea.
We have data-- students sending me pictures with the airport screening person.
So I love the excitement of thinking about this over Thanksgiving, but put it in your suitcase if you're going to take it home.
That's my why this matters.
So we're dissolving stuff today.
That's the topic.
How do things dissolve?
There's a citric powder, that citric acid that's going to make water more acidic.
And again, we're going to move into acids and bases next.
Today we're just talking about dissolution.
And so here's citric acid.
There it is.
Now over Thanksgiving, I thought I'd throw this in, because you might have sugar at the table.
You know to call it a c12h22o11.
Don't ask for the sugar.
If you want to add some sugar to something, ask for it by molecular name.
But the question is why do these things dissolve in the first place.
There is acetic acid, citric acid, there's sugar.
These are molecules that look like that.
Why do they dissolve in the first place?
And the answer is because of the things that we learned about bonding and about things like whether a molecule is polar or not.
Or in this case, whether you've got the possibility to hydrogen bond or not.
And so if you look at both of these, you can see that they both have a whole bunch of oh groups that look a whole lot like things we've seen that like to hydrogen bond.
You see those ohs on the end there, both for sugar, around the outside, and for citric acid.
And so what happens is if I put a sugar molecule into water, here's a picture of it.
There's a picture of it.
So there's sugar molecules there.
Now they start in a crystal of sugar-- you pour your sugar in, a teaspoon of sugar.
And it's in a solid, but then it goes off, and it breaks away into the water.
And those little ones are the water molecules.
Why?
Because the water was bonding to itself.
The water has dipoles-- dipole-dipole.
Remember that?
The water has hydrogen bonds, but the sugar does, too.
The sugar does, too.
And so the water sees this crystal of sugar, and it says well, hang on.
If you come out, we could bond together in much the same way that we're already bonding with each other.
The water molecules are already bonding with hydrogen dipole.
The sugar is like, well I've got hydrogen. I've got dipole.
And so it can break off, and it doesn't cost that much energy.
In fact, it gains energy by doing this.
They can gain.
And so this can actually be a lower energy state overall.
Now that's because of something that many of you have probably heard, and now we can put some bonding to it, but it's because like dissolves like.
Oh yeah.
That's technical.
That's technical because now we know what like means.
Like means a type of body.
like means a type of-- It's like water.
It has hydrogen bonding potential.
Therefore, it's able to dissolve in the water.
So water has-- well, what does water have?
It's got dipoles, so it can do dipole-dipole stuff.
It's got h-bonds.
Oh, everything has London.
Everything has London.
So if you've got those ingredients, if you've got that ability, if you've got those likes, then you might be able to dissolve.
Well, this is called a polar solvent because the molecules that make it up are polar.
They've got dipole.
Actually, and so we're very interested in understanding how things mix with other things and why they mix with other things.
Let's take a look at another example.
So here we have ethanol.
I keep on bringing up ethanol.
There might be some served, and if you're 21 or over, you can have some.
Otherwise, it's illegal.
Ethanol up there at the top.
Thank goodness, you will say, at your Thanksgiving table, it is not methoxymethane.
And because if it were, it would be the same chemical formula, but the oh-- the o would be in the middle of the two cs with the ch3s on the end, and you wouldn't be able to hydrogen bond it.
It would all just be vapor.
It'd be vapor.
No, but it's ethanol because it has hydrogen bonding capabilities.
But look at what happens if it's heptanol.
So all I've done now is I've taken the same end group, and I've given it more of a carbon chain.
How is that going to affect how it dissolves?
Given what we just talked about that like dissolve-- they both have hydrogen bond potential.
They both have hy-- but look, more and more of it, as you go from ethanol to heptanol, more and more and more of it is something else.
It's only London.
So what's going to happen now if I put this into water?
Well, we can see this going series.
Here's methanol, ethanol, propanol, butanol, pentanol, hexanol.
Heptanol is down at the bottom, and there's the water solubility.
We've got to write that down-- solubility.
So solubility is kind of what it sounds like-- the ability for a solute-- that's the thing we're dissolving-- to dissolve in a solvent.
That's the thing we're trying to dissolve it into.
That's the solubility.
And we'll be talking about the maximum solubility in just a few minutes, which is what we usually-- what's the solubility?
We often mean the maximum.
Well, that's what's listed here-- the maximum.
Methanol, ethanol miscible.
So you can put as much as you want, and it'll always keep mixing in with the water.
But now look, once you get to butanol, pentanol, hexanol, it starts going down.
You can't mix as much as you want.
In fact, it's less and less and less, and the reason we now know is because of what I just said.
As you go down, the hydrogen bond and dipole-dipole becomes less important because you've got more and more of the molecule that's only London.
And so it's less like the water environment, the bonding environment that the water liked with itself.
And so it has more trouble dissolving and being energetically favorable isolated by those water monitors.
By the way, does anybody know the origin of the word proof, speaking of ethanol?
If you buy a bottle of ethanol at the supermarket-- so there's gin or vodka or whatever, and it says it's something percent alcohol-- 20% alcohol, 40 proof.
Does anybody know where that comes from?
It's this.
It's just-- but what they did, they didn't know if you were making good alcohol back in the time.
And they said, well, I don't have all these detailed abilities to measure and quantify it, so I was going to light it on fire.
That is exactly what-- is this a good bottle of gin?
I don't know.
Let's light it on fire.
That's what they did.
And it turns out that if ethanol and water are at 50/50 mixture or more, it will light on fire.
Don't try that, please.
Don't do these experiments.
But so that meant is this a good bottle of gin?
Well if it's 50/50, I'm going to say it's a good bottle of gin.
So let's light it on fire.
Oh, it lit on fire.
That's 100% proof that it's good.
That is true.
That is the origin of the word proof.
It is proof that it's mixed well, and it's good stuff.
Dissolution-- dissolution-- well, what else can we try to dissolve?
We're dissolving alcohol.
We're going down to heptanol.
We're lucky it's not methoxymethane.
We talked about sugar.
So we've got to have a framework, by the way.
Solubility-- the ability for a solute to dissolve.
And now, there's a context here, right?
Let's suppose I'm dissolving sugar.
So sugar-- how are we going to write this dissolving down?
Well, sugar is c12h22o11, and if we put it in water, we could we could formally write the whole thing out.
We can write it out.
We can say I'm going to add water to it, and that's going to go to-- it's going to go to c12h22o11 plus more water.
But there's something very important that I'm leaving out, and when you think about dissolution, you've got to keep track.
What was it?
Was it a solid?
Was it a liquid?
Or was it something dissolved in a liquid?
So this started as a solid, so we put an s there.
This started as a liquid.
This is the dissolved thing, so we put an aq.
And the aq means aqueous.
It is in an aqueous solution.
It is a dissolved molecule.
And so here, this is a liquid.
Now the other thing that happens is-- well, the h2o is kind of there.
It's all over the place.
It's that solvent, and so we often leave it out because it's everywhere on both sides.
And so often, what you'll see, and we'll write more of these coming, is that you might write a dissolution of sugar as this.
Solid, and it just goes to something that is dissolved.
And you know that what happened there is sometimes you'll also see it like this.
What did you do on the arrow?
I added water.
That's what the arrow did.
We're just getting comfortable with writing these dissolution reactions.
Now what else can I dissolve?
Well here's another good example-- vitamin A. There's some vitamins you can have as much as you want, pretty much.
Why?
Because they're water-soluble.
And other vitamins are fat-soluble.
That means they don't like water as much as they like the hydrophobic molecules of fat cells.
And so they go into fatty tissue, where they don't come out.
That's why you not have unlimited amounts of vitamin A. That's not good for you.
But vitamin C-- no problem.
No problem, because it's going to dissolve in water.
And so your body can take in and get rid of as much of it as you want.
But if it's dissolved in fat cells, that's a different thing.
So vitamin A, vitamin C-- and you can see it right there from the chemistry.
This is it.
Look at all those oh groups in vitamin c.
Look at that.
Oh, that's going to love hydrogen bonding, and on vitamin A, you've just got the one all the way down there at the bottom.
It's hydrophobic.
By the way, in this class, we do not say the words hydrophobic interaction because that doesn't make sense.
There is no such thing as a hydrophobic interaction.
No, hydrophobic interaction is misused.
What it really means is that something that's hydrophobic likes itself more-- being together with itself-- more than it likes being in the water, or something hydrophilic.
That's what is meant, but there's no actual interaction there.
Hydrophobic, hydrophilic.
Well, those are other things you can think about dissolving.
You can also dissolve salt, and this is what we're going to use as examples.
And this is a picture of a salt crystal.
So we show the sugar, and here's salt. Now, so the thing about salt-- where's my sugar?
There's the sugar, and let's put salt underneath it.
The thing about salt, which is really just a general term for when you have an ionic solid that can be made from a mixture of acids and bases and dissolves oftentimes a little bit, at least in water.
So a salt or an ionic solid-- let's say for now-- this gives something different, because this breaks apart into positive and negative ions that they contain.
So in the case of sodium and chlorine-- sodium chloride-- well, that's going to dissociate.
If you pour salt in water, then here's the reaction for that.
You've got sodium chloride solid.
We put it in water.
That's going to go into ions.
So I put that in water, and it's different in the sense that it doesn't break down into the molecules that made up the sugar.
It has a particle of sugar that's got millions and millions of molecules in it.
Now they dissociate.
That's what the aq means.
Those are neutral.
Here, it's a solid, but you can understand this from looking at it and thinking about it.
Look at the way those water molecules are oriented.
They're pointing to the cl with their positivity, with positive charge.
And they're pointing to the sodium with the negative, and that's how they find happiness in water-- because they're ions, and water can be either way.
It can point its dipole in either-- and so it can actually bond.
Remember, the hydrogen has got a little positive charge because the oxygen took it.
And so that's what the-- it has a dipole, and that's able to turn around and bond and be happy with each of these ions.
That's why salt dissolves-- because it can find a happy place.
Other reasons exist outside of this class, like entropy, thermodynamics.
But this is how we're going to picture it as energetically happy.
It can become happy.
So we've dissolved some things.
We've got to start thinking about the thing that I mentioned before, which is how much can I dissolve?
How much can I dissolve?
And in that, it gets into this table here.
There it is.
So that's how much.
And you see it's listed there as moles per 100 grams.
And so that's one way to write it.
You could write it as-- it's often the max solubility is often what we're interested in.
And you could write it in moles per 100 grams.
You could write it in moles per liter.
Well that's something that we've already learned.
That's m form last Friday, the molarity.
Or it could be in grams.
Sometimes you'll see it in grams per liter.
So don't get bogged down by the units.
It's just how much of this stuff were you able to dissolve?
If we look at sugar-- here's sugar, here's salt-- how much sugar can dissolve?
I'm not going to cram it in.
So for sugar, the max is 1,800, so I'm going to do both those units-- 1,800 grams per liter.
And if you convert that, it's 5.26 moles per liter.
That's how much sugar you can dissolve in water.
By the way, I did a quick calculation, and that's 429 teaspoons.
That's 429 teaspoons.
I don't know what everybody is saying-- Coke has so much sugar.
Coke's got only 26 teaspoons per liter.
You could put a lot more in, if they wanted.
I'll go the other way.
I challenge them to go near the solubility limit.
They're not even close.
Too much sugar.
So salt-- you can also look at salt. For salt, what is it?
Salt is 359 grams per liter, and then that's it.
That's 6.14.
This is nacl.
It's interesting-- if you compare sodium chloride with sodium bromide-- so if you look at sodium bromide, it's going to be 8.9.
And sodium fluoride there's only going to be 1.
Why?
Because it comes right down to the picture.
I've got to make these happier.
I've got to make them happier.
If you've got the ions there, and the water likes it, but what if they really didn't have a strong ionic bond to start with?
You can imagine you might be able to get to a higher amount of dissociation.
Or what if they had a very strong ionic bond?
You might imagine that less of it-- you've got a lower maximum solubility.
So it all kind of goes together with the things that we've learned.
But what we're really talking about is what I need to tell you about next.
And that is this idea that we've reached a dynamic equilibrium.
We've reached a dynamic equilibrium.
So what does that mean?
Well, if it's unsaturated-- so if it's unsaturated, I'm way before this limit.
This is salt. That's the limit max.
Max.
What does it mean?
What does it mean to be below it?
Well, what does it mean to even have reached the maximum itself?
What it means is not that things aren't dissolving.
What it means is that things are reforming, recrystallizing at the same rate that they're dissolving.
That's what the maximum means, and that's an equilibrium.
And that's why we're going to talk about next.
So if it's unsaturated, there's a nice little molecular picture.
There's the thing you're dissolving, and look at them.
They're going away into solution.
Maybe it's salt breaking up into ions.
Maybe it's sugar.
They're going into solution and finding a happy place around the water.
But once in a while, one of them comes back.
You see that little particle there coming back.
That happens.
But much more, they're dissolving.
Then, you reach this point of saturation where it cannot-- where it's going to recrystallize, reform the solid at the same rate that you dissolve.
And that's the place where you can't dissolve anymore.
So what's really happening is that you have a solute plus a solvent, and it's not going one way as we've been writing it down.
It's going both ways.
So it's actually the solute plus the solvent goes like this.
That is what we've been talking about.
That's dissolution, and that's the predominant mechanism on the left before you've saturated.
But see, and that goes to some solution.
But see, you can also precipitate.
Precipitation.
And so at the saturated solution, you have-- if it's saturated, then that's another way of saying we've reached the max possible concentration of the solute.
Remember, the little brackets means concentration, molarity.
It means that these rates-- thinking back to Friday-- are the same.
It means these rates are the same.
This is a dynamic equilibrium.
And so that's what we need now the tools to talk about.
And so we're going to talk about three things-- the reaction quotient, the equilibrium constant k, chemical equilibrium, and then the solubility product.
Now if you think about-- I just said this is like the forward reaction is going in the same-- it's happening in the same rate as the reverse.
And so if you think about this as like a graph of concentrations-- so I'll just put here concentrations and time-- then maybe I have started-- I've put my salt. I pour a teaspoon of salt in water, and the salt is dissolving.
So it's going into the products.
It starts out at zero in solution, and then it becomes products.
At the same time, maybe I've started it up here, and these are the reactants-- Gesundheit-- that look like this-- Gesundheit-- and those would be the reactants.
So the concentrations of these things-- at a certain point, they're changing.
And then, at a certain point, they're not changing.
But they're still reactions happening back and forth like I just showed you.
It's a dynamic equilibrium.
Now what we do is we write down for some arbitrary reaction-- aa plus, so let's go back to this one.
But now, we're going to write it going both ways-- cc plus dd.
You can define the reaction quotient q-- that's the first thing-- as the concentrations c of the products-- c to the c, d to the d, over a to the a, b to the b.
Please do not confuse this with the rates.
Yeah.
I've been talking about the rates being equal.
That's not from the rates.
Remember, the exponents of the rate law come from experimental measurements.
The reaction quotient comes from the stoichiometric coefficients.
Why?
These exponents here have to do literally with probabilities of reaction, of reacting.
It has to do with the fact that if I've got two of c, then you multiply the amount of c times another amount of c in the same volume.
It has to do with probabilities of reacting.
That's where you get those exponents, and they're directly from the stoichiometric coefficients.
They do not need to be experimentally measured.
Once you have this, you have the reaction quotient.
Now, why is this useful, this reaction quotient?
Well, it's useful because there is a very special place where now it doesn't change anymore.
These concentrations aren't changing.
Reactions are still happening, but the concentrations aren't changing-- no change.
If there is no change, then-- Gesundheit-- then q isn't changing because it's just a ratio of concentrations.
It's a ratio of concentrations equal to this whole thing, not just the top line.
The reaction quotient is related to the concentrations.
So if the concentration zone changes even if reactions are happening still, it's a constant.
So at this point, q equals a constant, and that is an equilibrium constant.
So we'll call it keq.
It's a dynamic equilibrium.
k represents a dynamic equilibrium.
And what's really useful about k is that for a given reaction, k is a constant.
I just said it.
But it doesn't matter-- if we're-- it depends on temperature.
But if I keep the temperature fixed, k won't change.
It's a constant.
It's the constant that tells me where equilibrium is.
It's the constant that tells me where equilibrium is.
Now there's one more thing that we've got to write down-- that third one there.
I'm going to put it on here.
So k is an equilibrium constant.
That's a general constant.
But now, I'm going to turn my attention from any old reaction-- my k is actually very general concept for equilibrium.
We are in this class going to use it for solubility.
That's how we are using the equilibrium constant.
And so the ksp is the solubility product, and it's an equilibrium constant.
Equilibrium constant that is related to solubility.
It is literally the product of solubilities in equilibrium.
That is literally what it means.
So we're going to do some examples to see that.
It's an equilibrium-- so it's a constant of equilibrium.
And it's called-- Gesundheit-- it's called the solubility product, because it's related to the equilibrium of dissolving something, dissolving some solute in a solvent.
Now we're going to do examples with salts.
So we're going to be dissolving salts and talking about equilibrium constants in that dissolution, otherwise known as the solubility product.
It's just an equilibrium constant.
There are many.
This is the one we're going to care about, and we're going to do it for salts.
And so one of the things you've got to-- so nacl-- that was kind of easy.
That became a cation and an anion in solution.
But salts can be made of many, many different types of cations, and there's lists that go on and on and on.
Here are some, and they get named.
So here's some of the anions.
Oh, chlorine-- we just did that one.
Bromine-- you can also have a carbonate.
You could have chromates, hydroxides, oxalates, sulfates, sulfides, and the list goes on and on and on.
There are many, many of these.
They don't have to be a single atom.
It can be a part of a salt that breaks off and has a negative charge.
That's an anion, and here are some of the cations-- sodium, potassium, calcium.
And you know about these things.
We've been talking about these things.
And you know if it's sodium, then you know it's only going to lose-- it's only going to be na+.
It doesn't have a second weakly-bonded electron to lose.
So it's really only going to be na+.
But then you get-- and calcium is going to be calcium 2+.
It's got two, magnesium two.
And then transition metals are a little more complicated.
They can be a little more complicated.
Iron can be 2+ or 3+.
It depends, and on and on and on for those.
And so we'll do a few examples.
We'll do a few examples.
We'll get into the mood.
And I want to do it-- first, I'm going to do an example that's actually a classic example, because it doesn't dissolve very well, but it's silver chloride.
Now sodium chloride is-- sodium chloride goes all the way to the point where it basically dissolves so well.
So you can imagine the equilibrium for sodium chloride goes way to the right.
But for silver chloride, it's different.
For silver chloride, it's different.
So we're going to look at this curve.
And I'll talk about this curve.
Don't worry.
We're coming to it.
We are coming to it.
Let me start in the middle again.
Because what the equilibrium constant does, given the definition that I have just hidden, unfortunately, is it allows us to go back and forth.
If I know the equilibrium constant, then I can get how much one of these ions is soluble.
I can get the maximum solubility of it.
So let's take an example.
So here's a question.
How much agcl dissolves in 1 liter of water?
That's such a good question.
I don't know.
But I do know that the solubility product that this equilibrium constant for sodium chloride is 1.7 times 10 to the minus 10th.
Now if I write down the dissolution reaction, sodium chloride solid is going to go to-- sorry, sodium silver-- silver plus in solution.
So we put the aqueous plus chlorine minus in solution.
And it is going to go back and forth.
And so the ksp for this is going to equal-- I'm going to go with the equation that I wrote down-- the concentration of silver to the 1 times the concentration of chlorine minus-- these are ions-- divided by the concentration of silver chloride solid.
Now we get to another thing.
Remember, I got rid of water, because water is everywhere.
It's the solvent.
So you don't need to put it into these equilibrium calculations.
Well, it's the same thing with the solid.
So if I think about this a little bit, this equilibrium is between dissolved.
It's between the ions that are dissolving.
But there from this reaction, from the way it's written there, I've got a solid form of silver chloride.
So this is not-- so this is the concentration of solid silver chloride in the solid.
This is just a constant.
That's not going to change.
That's not going to change.
Common misunderstanding.
It doesn't change.
So we get rid of it, because it gets folded into the constant.
That's always the same.
The concentration of this is always the same.
And so we get rid of it.
So now we have the ksp is equal to, in this case, ag+ times cl-.
Talk about solubility product.
Solubilities concentrate.
The solubility is-- when I add this in, it reaches some equilibrium where they're dissolving and reforming.
I wanted to know at what point that is, what concentrations is that going to be.
This is them.
So solubility product-- right there.
I used the definition of equilibrium constant.
And now I can use this reaction.
I can use this reaction because the stoichiometric coefficients tell me-- they tell me both what the exponents there are-- 1-- but they also tell me the relative molar amounts of these things.
It's the same.
So if x equals the concentration of silver plus, then it must also equal the concentration of cl minus.
If you just let x equal the concentration, that comes from the reaction.
1 in front of silver, 1 in front of chlorine.
And so that means now that you can solve it because x squared is equal to 1.7 times 10 to the minus 10th, and x equals 1.3 times 10 to the minus fifth, m, and that equals these concentrations.
That equals the concentration of silver plus.
So that is how much, in equilibrium-- this is an equilibrium constant-- that is how much you will get of these ions dissolved in water.
Now there is a nice way to set this up that is often taught in high school.
I like it.
I think it's a good way to keep track.
And so if you were wanting to keep track, and you like tables, there's something called the ICE table.
And in this case, it's a little bit boring, but as you'll see, it can actually wind up being pretty useful, especially when we add other ions, which are going to do soon.
So ICE is initial change equilibrium.
Initial change equilibrium.
It's just a way of keeping track of what I just did, because I started with some amount of solid, and I had zero.
I put it into water.
I had zero, but then I added 1.3 times 10 to the minus fifth, and I added 1.3 times 10 to the minus fifth.
And so my equilibrium is a little less, a little less solid.
And over here, it's just these numbers.
That was indeed boring, but have no fear.
It becomes helpful.
It becomes helpful because what we're going to do next-- not right now-- but on Wednesday, what we're going to do is we're going to mess with this equilibrium.
Oh, equilibrium, I'm going to throw you off.
And equilibrium is saying, no you're not, because I'm equilibrium, and I will always be found.
And that's called Le Chatelier's Principle, and what that does is it allows us to really go back, and we get to actually add a bunch of chlorine ions to this, and we're going to see what happens to this balance.
It will find equilibrium.
And we have the tools now to know how to find it.
That's that green curve there.
This is what we're going to work with.
There's the concentration of chlorine.
There's a concentration of silver in ions.
You see the dissolved chlorine on the x-axis, dissolved silver on the y-axis.
And look at that.
I've got that point b there that is where these things have found equilibrium when it's not messed with.
Now the thing is, if you were a reaction happening, and you hadn't gotten to equilibrium yet-- so I hadn't added much silver chloride-- if that was the case, then you might be at point d.
Notice that the reaction quotient, q, is less than the equilibrium constant.
q is less.
That means it's going to be driven to dissolve more than it precipitates until it gets to point B on this curve.
You could also be way, way out with a much higher q point, e, and you know it has to find equilibrium.
It's going to find equilibrium, which is what we just calculated.
Those are the concentrations of equilibrium.
It has to get back to point B. It's going to do it by precipitating out solid.
It's gone too far.
But you can see that equilibrium can also be had and will be had.
It shall be had.
Because k never changes if you fix the temperature.
k is the same.
So I can mess with this in other ways.
I can go and add a whole bunch of chlorine, and it will come back down onto that green curve.
It will come back down onto that green curve, which is the curve that guarantees k is the same constant.
That's what that green curve is.
And there's one last point I'm going to make today, and that is units.
Units of k depend-- it was like the rate law.
It depends on the reaction.
Look, a concentration is a concentration.
That was deep.
Units are capital M moles per liter.
But that means that k-- k is two of those multiplied together.
So in this case, k has units of m squared.
It must, or it wouldn't work.
k can have units of m cubed.
We will pick up here on Wednesday, and we'll cover more of this, more examples before we move on to acids and bases.