Flash and JavaScript are required for this feature.
Download the video from Internet Archive.
Description: This lecture describes how atoms of glasses or “amorphous materials” are randomly arranged in a non-repeating structure.
Instructor: Jeffrey C. Grossman
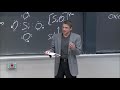
Lecture 25: Introduction to...
Today, we're going to just start by talking about what glass is and what its properties are.
But before we do, this came in-- we've been talking about defects, OK.
And vacancies, point defects, and then on Wednesday, we did line defects.
Remember those?
Right, and they create these planes that come in.
They're slip planes that allow atoms to slide across each other that allows you to plasticly deform a material.
And so OK, but with regards to vacancies, I got this by email.
I'm at the Heathrow Airport in London, and even this aerial sculpture has vacancy point defects in its pattern.
Look at that.
There you go.
And there you go.
This is a beautiful thing, right?
Thank you, Sophia.
And notice that she's looking at it with her periodic table, of course, right.
That's a no-brainer.
But even such structures can't escape the fact that you always have vacancies.
That was cool.
OK, now where were we?
Right, so we're going to talk about glass.
Now, this is glass, and I'll start with just how cool glass is.
But this is also not just-- this isn't all that glass is.
And I want to make sure we get that by the end of this lecture.
This is not the only thing that glass means.
But this is what we think of.
So here's a really cool video.
OK.
No and yes.
[VIDEO PLAYBACK]
There they are.
Look at that molten [INAUDIBLE] and it's-- oh, he just added something.
We'll talk about that.
Oh, and now he's mixing it.
- Table man carefully mixes this glass [INAUDIBLE]..
Once the glass is blended to his specifications-- OK, there it is.
Is it a liquid?
Is it a solid?
- [INAUDIBLE]
And then he just puts it through a little roller.
- That will mash that glass flat [INAUDIBLE]
Mash it flat, and there is your piece of glass.
OK, and we'll see another one.
I'll show you another video of a larger scale-- don't keep going.
[END PLAYBACK]
OK.
So what was it that they were just mixing?
Why does it have the properties that it has?
Why can they just take this thing that's not really liquid and not really solid, it's some viscous thing, and roll it through, and then it comes into this really beautiful sheet that we all call glass?
And it all has to do with what I showed you before, and what we've been talking about, which is order or disorder.
And so we did this already.
We covered, OK, a solid that which is dimensionally stable.
That's cool.
Classification, oh ordered, regular, long-range order, BCC, FCC.
All right.
OK, long-range.
Remember, we said it just keeps going and going and going.
And then we say, well, it may be once in a while, once every 1,000, 10,000, 100,000, there's a vacancy.
OK, so we started messing with it.
But it still had this long range-- gesundheit-- order.
And so they were called crystalline, crystalline with defects, maybe, but still crystalline.
That is not what glass is.
Glass is disordered.
And so that is the topic of today and next Wednesday.
What happens when you go from an ordered structure, like this, oh maybe it's got some-- oh these are 2D defects, right, the whole things are kind of-- so it's poly crystalline, but it's still kind of crystalline, and it's very ordered, to totally disordered.
All right.
And so a disordered solid looks random, certainly over long range.
There might be short range order.
There might be some short range order, but over a long range, it's going to be disordered.
It's going to look random.
This is called amorphous as opposed to crystalline.
This is also called glass.
This is also called glass.
So one of the first things we've got, this is really important, glass is not just the window, or the thing on your phone that keeps cracking.
No.
Glass is equal to an amorphous solid.
Well, so it's bigger.
It's broader.
It's bolder.
You can have glass metal, metallic glass.
It's a thing.
Any solid that doesn't have long range order that's amorphous is a glass.
OK.
Now in this class we will use SiO2 as-- so what we're going to do is we're going to talk about glass with an example.
We will use the kind that winds up being in the windshield of your car or in the window of your house and on your phone.
And that is called quartz.
So the chemistry that we're going to use is quartz, or SiO2 OK, and what we're going to talk about is how processing effects that chemistry, affects the-- sorry, the solid that forms.
So processing.
And there is lots of processing parameters and we can talk about.
What I want to focus on today is the cooling, the cooling rate in particular.
So that's going to be the focus today.
We'll talk about the chemistry.
We'll talk about the processing.
And we'll talk about how those go back and forth to create glass.
Now, OK, what am I starting with?
I'm starting with a crystal.
This is quartz.
This is how you see quartz.
Now, quartz is this beautiful, beautiful crystal.
And it is ordered.
That's why I'm saying the word crystal.
I'm not saying the word glass.
All right.
This is an ordered solid.
It's not cubic.
So it's not one of the ones we've learned.
But it's still periodically repeating.
All right.
And so it's a crystal.
It's got long range order.
It's called quartz.
It's SiO2 So I'm going to hand this out so you can just see it.
And that's what I want to start, I want to talk about, is that crystal, OK, because that's what we're going to be messing up and creating short-range.
We're going to go from long-range to short-range.
OK, so what is it?
Well, let's see.
In this class, how do we think about chemistry?
Well you can think about it now in different ways.
Let's pick Lewis.
All right, OK.
So I say, OK, I got silicon, silicon, silicon, if I think about silicon as it's Lewis, you know, dots, then it's got four.
It's got four valence electrons ready to bond.
You can see that now if I have oxygen, what does oxygen look like?
So oxygen has six.
I'm going to put those like that.
So oxygen has six.
Maybe it's got a couple lone pairs, which has six valence electrons, not four.
Now so if I had-- so when you look at this, you think, you know, silicon looks like it wants four bonds when you look at it.
And in fact, we know that the crystal in silicon as we've talked about-- gesundheit-- is tetrahedral because of this.
Right, it's tetrahedral.
Remember, it's a two atom basis FCC lattice which is is diamond.
But what if I just look at it with oxygen, come at this with oxygen and say, well, you know, I want to have four bonds.
One way to do that would be to add four oxygens.
So what if I did this?
What if I did silicon and oxygen, and I did plus 4 times the oxygen.
Well, then you could see, what could you do?
You could do this, OK, and this and this and this.
But now, OK, the oxygens, how would they be left?
They'd be left like this.
And you'll see why I'm focusing on this SiO4 group in a minute.
That's what I got.
Now, OK, if I go back in and I put my Lewis cap on, silicon is very happy it's obeying octet, but the oxygens aren't quite there.
So they need one more electron, one more electron.
I'm going to make it a little bolder because I've added it.
All right.
So I've added an electron to each of those.
So if I had SiO4, and I added 4-- if I had SiO4, and I added four electrons to it, I get a really nice stable Lewis structure.
Right.
That's called a silicate.
That's a silicate group.
SiO4.
And I need those four electrons, as you can see just from looking at the simple dot diagram.
I need those four electrons to make it happy.
OK.
By the way, just speaking of naming, OK, so let's see silicate, right silica, SiO2, we can talk about, so what we do is when we have oxide, silicon oxide, just in terms of naming, you know, like if you have an oxide, often, you kind of add an a, right, so like silicon went from SiO2, we call it silica.
So in Al2O3, let's see, Al2O3 is also an oxide, right?
And so we called that-- this is something we've already done, alumina.
All right, and so on, except for sometimes.
And so sometimes, like for example, if you do calcium oxide, well, that's called lime.
It's called lime, calcium oxide.
Well, what about sodium?
Well, sodium, if you do sodium, you're going to need it I'm assuming the oxygen might break off and become to-- so you've got to think about the charge again.
So Na20 would be the one that works there.
All right, and this is sodium oxide.
But see, sodium oxide, but sometimes, it's called soda, because-- and this is an extra step, because soda comes from calcium from-- let's see, Na2O from-- how do you make it?
You make it from Na2CO3, which is actually what is soda?
Sodium carbonate.
But because we make Na2O, that oxide from something that we call soda, sometimes people just call in Na2O soda, it's not.
But sometimes you see that.
So I just want to kind of make you aware some of these names.
Why am I writing it there?
We're coming back to that.
We're going to use those later.
That's partly why I want them to be on the board, and also tell you about the names.
So Na2O is sodium oxide.
But it's made from sodium carbonate, which is soda.
OK.
All right.
Now, OK, now, here's the thing.
That's an isolated molecule.
Look at what I'm passing around here.
Oh, I wanted to tell you about the window.
I almost forgot.
In this class, in this class, we don't go around saying that windows are thicker on the bottom, than on the top, because glass flows like a liquid.
No.
That is not true.
That is not true.
That may be true down the street.
That is not true here.
We do the calculation.
Glass is not a liquid, not once it's cooled down into a solid.
It's a solid.
If you do the calculation, and it's a centimeter thick, a window pane, for that piece of glass, under normal diffusion and temperature conditions for that piece of glass at the bottom to become thicker by one nanometer would take 10 billion years.
OK.
So no, it's not flowing under its own weight in a window.
What happened?
What happened is people didn't know how to make glass uniformly way back when, and so they came out un-uniform, non uniform.
What's easier?
To install it with the heavier side up or the heavier side down?
It's easier to install it if it's heavier on the bottom.
Class doesn't flow.
OK.
All right.
I wanted to get that done early.
Yeah.
Oh, here we go.
OK, so this is quartz.
This is crystalline silica.
This is groups of SiO2, not SiO4.
This was how we made a molecule happy.
But watch what happens now when I go from that picture to SiO2.
So now, I've got these-- let's suppose I've got these molecules of-- and I'm just going to not draw it 3D, but I'm going to draw it 2D, and OK, there's-- gesundheit-- there's all these charges there.
So now these are these molecules, these silicate groups.
And they're kind of happy, and they're on their own.
But now look what happens here.
See, those two oxygen, what if instead of each of these being separate, they came together?
All right.
Well, you can see that-- you see, if they shared the same oxygen here, if they shared the same oxygen here, then that oxygen is actually now Lewis happy without the extra charge.
So this oxygen, this bridge, oxygen, doesn't need that extra electron to be happy.
What does to be happy mean?
Well, we know what that means.
And if we're talking about Lewis and speaking in those terms, it means you've got your octet.
All right.
So now the bridge oxygen-- oh, but that is what I'm passing around.
That is what quartz is.
It's all the oxygen is happy without needing the extra charge, because they are all bridged.
So that's what we've done.
We've made silica by thinking about it in terms of these silicate groups, OK, and just bridging them all together.
And I can't draw it.
But you have it there, and 3D models are the easiest way to see it.
And they all act as bridges between silicate groups.
OK.
So why am I-- why do I keep this silicate group?
So a lot of times if you look up the structure, and this is the 3D structure, you see every single oxygen is a bridge.
Every single oxygen is a bridge.
And it's nice to think about it in terms of the silicate groups.
Why?
Why did I start with that?
I started with that because these groups are very strong.
They stay together.
And they kind of act as building blocks for the glass and for the quartz.
And you now know those are two different things, quartz is silicate groups ordered in a crystal.
Glass is what we're going to get to.
It's when they're disordered.
OK.
And why is that?
Well, because these oxygen, you see the silica, the tetrahedron with the silicon atom and those oxygens is pretty robust.
But the bond between the oxygens is-- well, there's a whole lot of rotation that can happen here.
You see that?
That thing can rotate around.
So that's a good way to picture this.
That's a good way to picture quartz is these silicate groups that are bonded together through these bridge oxygens, but they have a lot of rotational and distortion relative one with respect to one another, that is possible.
So I take those silicate groups, OK.
Here they are up top, and I bring them together, and I make a perfect crystal.
That is what's being passed around.
But look, because there's so much possibility for rotation and distortion, maybe that didn't happen.
Maybe as they start to form or they find each other, and they start bridging, maybe they didn't quite make it.
And you can imagine these things are kind of bulky groups coming together and twisting around.
You missed the mark, it might be hard to get back.
So how does one happen over the other?
All right, that's the crystal.
That's the not crystal, the glass.
And it depends on a couple of things.
And that's what I want to talk about next.
It depends on the temperature and the cooling.
Depends on the temperature and the cooling.
So how does temperature come in?
So temperature comes in because temperature makes everything vibrate.
Right.
It gives these atoms and these groups kinetic energy.
OK.
So let's look at that.
So if we were to plot, for example, temperature versus the volume per mole, so this is, you know, for a given number of these atoms, much volume do they take?
Well, you can imagine that that is actually related to how much energy they have.
All right.
So like if I had like a crystal, you know, maybe I'll start it here.
And if I have a crystal, you know, and I increase the temperature a little bit, maybe they're going to move more.
And as they move more, they need more room.
And this is just a simple-- you can just think of like a simple atom, connected to another on a spring.
And more kinetic energy, more temperature, it goes further.
And now you're all thinking, well, wait a second, doesn't it go in as far as it goes out?
Why?
Does it need more volume?
Well, that is because of what we have already talked about, which is this energy curve between two atoms, or two silicate groups.
This potential energy curve that plots the energy between those two groups and, say, the distance between them, has something very important about it, has something very important.
This is the spring.
OK, so I'm in there, and I'm at T equals 0 here.
That would be like T equals 0.
The absolute ground state, the place where everything is just in its minimum energy, but now I start making things move.
All right, I put kinetic energy into it, I increase the temperature, what happens?
The energy goes up.
But look, it doesn't go up.
It goes like this.
The average-- I'm a little bit higher in energy, right, I'm a little bit higher in energy.
So where I am is there, on average.
Now I'm a little bit higher in energy, and so where I am is there, and now I'm a little bit higher, and where I am is there.
This is what happens to the average.
That's why solids expand.
It's because of the asymmetry in this potential energy curve, which we have already talked about.
It's because of the asymmetry.
You see.
This is going out differently than this goes up.
That's why you have thermal expansion.
All right.
Things are vibrating.
But more often than not, they're going to be farther apart.
And so this happens.
And so this happens.
So we've just understood this from an atomic-- so this would be like if I had a crystal, and I'm going to save three letters and write xtal.
That's so efficient.
And then I keep going.
And by the way, the slope of this is called slope is called the thermal expansion coefficient.
Thermal expansion.
That is the definition of the thermal expansion coefficient.
Sometimes we use alpha.
That's the slope of this line.
It's the volume per mole divided by-- over the temperature, the change in the volume or the change in temperature.
It's the thermal expansion coefficient of the material.
OK, so I'm adding temperature, and I'm expanding and I'm expanding and then I get to this point.
I get to this point.
This point here is where everything changes.
Everything changes.
That's the melting point of the solid.
That's the melting point.
Now at the melting point, as we know, the whole thing goes through a transition.
It goes through a phase transition.
And it becomes a liquid.
And the thermal expansion of the liquid is different than the solid, because those, now, you think about those molecules are not rigidly bonded to each other anymore.
They're more weakly bonded, and they're moving around with a lot of kinetic energy.
And so when they get more kinetic energy, they can expand even more.
That's why those slopes are different.
But as we know, there's also a big volume change.
There's a sudden volume change.
In going from a solid to a liquid, there's a sudden volume change.
And it would be the same thing if I went back the other way.
Right, I'm cooling it down and cooling it down, and all of a sudden, I get to the solidification temperature of the material, and I become a crystal.
Except when I don't.
Except when I don't.
And when I don't, that's when I become a glass.
And that's what we have to talk about now is, how do we go from this very simple picture of being a glass-- being a liquid or being a solid, a crystalline solid, how do we go from that to being a glass?
And to explain this, to start, I want to show you something really cool that can happen.
That actually wasn't an intentional pun.
But it has to do with cooling, and it's really cool, because sometimes, if you cool a liquid down, it doesn't solidify right there.
Then that's called super cooling.
So you can actually cool the liquid down like that, and have it stay a liquid.
That's called super cooling.
By the way, so this is called super cooling.
By the way, you can do that with water.
And so I want to show you like one of the coolest things you could do tonight.
It's a Friday night.
You're going to have guests over, or you'll be out at that same restaurant that knows about you, because you asked them already all about the candle and the oxygen.
And you always come with your periodic table, and this time, you sit down and you say I don't want water.
Do you want sparkling or regular?
I want super cooled.
This is what you're going to get.
This is what they'll bring you.
They should.
If it's a good restaurant, this is what they would bring you.
You know, so here's who is supercooled water being poured.
This is what you should see in your glass.
Now, that is a liquid that is stable, for now, below its melting point, below its solidification point.
So what happens is as soon as it hits the glass, it's like, whoa, wait a second, I am supposed to be a crystal, and it immediately solidifies.
All right, so it immediately went like this.
Now, by the way, it's not that hard to make supercooled water.
If anybody's interested, I'd be happy to tell you how to do it.
You can't just put bottles in a freezer.
That won't work.
But it's not that hard to do.
Now, OK, back and forth, melting point, supercooled-- oh, it's playing again.
And it starts freezing.
You can also take a bottle of supercooled water and just go like this.
It's liquid inside and just tap it, and the whole thing freezes instantly.
It's really cool.
It's really cool.
OK.
OK.
Now, why am I talking about this?
Because you can super cool and go down.
You could go below that transition point and go and go down, like I just did there and become a crystal right away, but you could also not.
You could also become a solid, so you could become a crystal, or you could also become a solid.
And I'm going to need another plot to do this.
And you could become a solid right where you were.
You might not go down to the crystal curve, but you might start a new curve.
And that will be a glass.
And so we're going to draw this again and show you a glass, but this is what we need is this framework to understand when a glass forms.
So this was our picture, crystalline, crystalline, glass, amorphous equals glass.
When does it form?
Now I think the best-- I'll show you that one in a second.
I think the best way to think about it is let's draw the plot again, and I think the best way to think about it is an analogy that has been used before, and let's see-- and this is temperature.
This is volume per mole.
By the way, that could be energy, or enthalpy.
Yeah, because as the atoms aren't packed in as well, we know this, right, as they're not packed in as well, they're also going to be higher in energy.
Right, the bonding won't be as strong.
But I think that one of the best analogies to think about is so I'm coming in as a liquid, so I'm now a liquid.
And here's my melting point for the crystal, for the crystal.
But I went past it.
And I went past it, and now, instead of-- I'm just going to draw this out here.
There's the crystal.
Instead of going down to become a crystal, suddenly or super cooling, and then becoming a crystal, no, I super cool, and then I just become a solid.
And then I just become a solid.
OK.
Now that-- this is a glass.
You know that it's a solid.
It's got the same slope as the crystal.
The thermal expansion of this thing is the same as the crystal or very similar.
So that's enough to tell you this is a solid, right?
It should be a good indicator.
It should have been a nice straight line just for now.
OK.
Yeah, but it didn't get-- those silicate blocks didn't find the lattice.
They didn't find the lattice.
As you're cool-- here, they've got all this freedom.
I mean, they're a liquid, silicate, happy.
Maybe it's an even higher temperature, and the silicons are even dissociated from the oxygen.
Let's just assume they're in these silicate groups, and they're floating around in a liquid, and I start cooling it.
I think one of the best analogies that I've heard is that of musical chairs.
How many of you have played musical chairs?
OK, you've got to fix this tonight.
For those of you who didn't raise your hand, that's going on the menu tonight, because it's a really fun game.
With supercooled water, of course.
You line up some chairs, and you get around in a group and you walk around it as the music plays and then the music stops and everybody has to find a chair, but there's a chair missing.
So you've got to go fast, or you might not get to a chair.
Well, you see the silicate, let's put it below here, the silicates are you.
You are the silicates.
People equals silicate.
And the chair equal lattice sites.
And now you can really feel it.
You can really feel it, because the speed around the chairs.
How fast are you moving?
Right.
So the speed, well, if it's speed around the chair, OK, if that's fast, then it's, you know, high mobility, so let's see, speed around chair, let's do this.
High mobility, maybe you'd find, you know, find lattice sites easier, or faster, at least, but see, if you have a high 1 over mobility-- by the way, that's also viscosity, if you have a high 1 over mobility.
So if you have a high viscosity, [INAUDIBLE]..
High 1 over mobility, high viscosity, then it's slow.
And that's how you have a higher chance of forming glass.
So I'm going to talk about what would make a glass form.
If you're just walking around slowly, like this, everyone else is running, but maybe you're all walking slowly, and now the music stops, and I'm like, yeah, I'll take my time, it's going to be hard to get to the open lattice site.
I might just get stuck.
OK, so what else?
Well, OK, so how about the arrangement?
So the chair arrangement, that has to do with the crystal complexity.
And you can imagine that if the chairs are arranged in a straight line, and you're going around the chairs, and it's is kind of an easy loop, right, now I don't have to concentrate too much on where I'm going, and it's kind of-- there's nothing really blocking me, I hope, but what if those chairs were arranged in a really complicated way.
So how hard is it to get around these chairs?
How hard is it to find them?
How complex is the lattice?
And you can imagine that it is higher.
So let's see.
More-- I'll just draw an arrow.
Higher would lead to glass.
OK, so it fits.
Higher complexity of the lattice.
It might be harder to find the lattice sites in this musical chairs.
OK, one more, and I'll put it here, so that it's close by, because I've got no more room on that board.
The third one is how fast you stop the music.
So this would be like 1, 2, and then 3.
How fast do we stop music?
OK.
So you can imagine if I, you know, if I slowly were playing musical chairs, and I am walking around, and I slowly fade the music, all right, well, then you got lots of time.
You got lots of time, but what if I just stop it.
Now, everybody's scrambling.
So how fast do you stop the music is the cooling rate.
It's how quickly you cool this material.
It's how fast you stop the music, and you can imagine that if it's faster, faster, you are more likely to form a glass.
You're more likely to form a glass, and so we go back to our picture.
Well, maybe I'll draw one over here, because I need more room, and I don't want to block what's there.
We go back to our picture and here it is, drawn out for you.
You see that is-- OK, there is the liquid line, coming in, volume, OK, enthalpy, visage, and we'll keep thinking about it as volume.
The liquid line comes in.
You're cooling it down.
There's the thermal expansion.
It's reducing, reducing, and now I get to the melting temperature, and I become a crystal.
That's if it can become a crystal, but all of these factors, now, matter.
The melting point of the crystal is always the same number.
It's always the same number.
The melting point, where the crystal melts, because the crystal is always the same.
Right.
Now, where that melts is always the same temperature.
But notice now, what have I done?
I've super cooled the liquid down, and on a, I've got a glass that looks like this.
These are glasses.
And on b, I've got a glass that looks like that.
Those are different.
And they have different solidification temperatures.
And what do we call it?
We have a special name for it, too.
We have a special name for it, because it's not crystallizing.
Right, so if this is the volume per mole, and this is the temperature, it's not crystallizing.
No.
What is it doing?
So that would be the melting point.
It is turning into a glass, and so we call this the glass transition temperature.
It's a glass transition.
What that means is it is still becoming-- gesundheit-- a solid, but it's an amorphous solid.
It's not a crystal.
Why did it become an amorphous solid?
Well, maybe I cooled it really fast.
Or maybe it's a really complex structure, right?
Or maybe the viscosity of the material is very high, and it just-- as I'm cooling it down, it just couldn't find the crystal lattice, which is all the way down here.
And you can see from this, that you know, imagine I take the extreme limit, and I got my liquid of my silicate groups, and right as I get below here, I'm like you're all frozen.
I'd literally-- I'd make a glass right here, right up there, all right.
So I can make glasses that are different from each other.
And you can see that the volume per mole is increasing as I go up, and you can understand that, because look, I took a glass.
I didn't let-- I took a liquid here.
This is the liquid.
I didn't let any of it find crystals sites.
It's a liquid.
But I said, ha, you're frozen.
And it became frozen and stuck at a high volume per mole.
Because it's totally random and amorphous.
It's coming right from all those random liquid degrees of freedom are just frozen in.
Yeah, but now, I cool it slower.
Maybe that's what happened here, right?
And I give it more time.
And I give it more time to find some of those lattice sites, and it finds some lattice sites, but then it became a glass at this temperature, the glass transition temperature, and everything else is frozen in.
So if I label this, so let's label it the same as there, if I label a as this one and b as this one, and this is the crystal, nope, the crystal would have to go all the way up to here.
There we go.
Right.
So if that's a, that's glass a, and that's glass b, then which one did I cool faster?
Well, I had to call this one slower-- if all I changed between them is cooling rates, then this one is cooled faster.
So let me write it here.
a cooled slower than b.
Right.
Because I get-- and that's this one here.
How fast did you stop the music?
Right.
It's the cooling rate.
OK, so this plot, this volume versus temperature really allows us to understand how glass forms, or at least we can plot, you know, what happened, and then try to understand it.
And if it did get all the way to the crystal, then maybe it means that it's not very viscous as a liquid, it doesn't have a complex lattice site, and maybe it didn't even matter how quickly-- oh, metals.
But that's why it's hard to make glasses out of metals.
Because metals have an easy time finding their lattice sites.
Metals as liquids typically aren't very viscous.
And so even if you cool it faster, it's got time.
The musical chairs of metals is easy.
It's an easy game.
That's why the silicates never played musical chairs with metals.
But I can still do it, and the way you make a glass out of a metal, the way you make a metallic glass, which is a really cool material is you freeze it really quickly.
You will lower the temperature as quickly as you can, really, really fast, and you freeze in the disorder, and you make a metallic glass.
OK, so those are the two curves I just talked about there.
That's the glass curve.
This would be the crystal curve.
OK, two different cooling rates on here.
OK, now, here's a picture of float glass.
This is the kind of glass-- we're going to move from understanding what glass is and understanding in terms of this temperature versus volume plot and amorphous solids, and we're going to move towards understanding how to modify it, how to control it, because we do a lot of that.
Here's an example of a factor right-- I like this.
This is flow glass.
So this is like when you buy, you know, your windows, or you know, a lot of times, when you need a very clear, beautiful piece of glass, you go up to very high temperatures.
All right, so you're taking those silicates.
You make them into a liquid.
Here he is, measuring the temperature.
I don't know what he's doing actually, whatever.
Oh and this isn't showing up very well.
And there's no volume, so double-- OK, there it is.
So there's the liquid glass.
Why is it called float glass?
[VIDEO PLAYBACK]
[INTERPOSING VOICES]
- The process is monitored continuously by technicians to ensure quality.
But because it floats on top of another liquid.
That's how you get the glass because so incredibly smooth.
- And ensure uniformity-- And there it is coming out.
There's a nice wide piece of glass coming out, and what he says at the end of this, there it is coming out.
[END PLAYBACK]
They can do kilometers and kilometers per day of this float glass.
You float it over molten tin.
The tin acts like this really nice smooth surface that you put the glass on top of.
So one liquid on top of another makes a very nice smooth interface.
And what he talks about, which is what I wanted to capture, is how important that cooling is.
So the line has to go in a very particular way after it comes out, as you cool it, and because it's all about the cooling.
As you come all the way back down to here, room temperature, right, or as I-- maybe as I went through this transition, what's happening inside?
Am I creating a lot of stress?
We just learned about stress.
Am I creating a lot of stress?
Right, or is it going to cool in a nice way?
Are there going to be cracks that form?
So that is one of the most important processing parameters.
What we'll learn next week is that chemistry is also a really important pressing parameter.
And that's why you have such good phone screens.
Actually, you don't really, because they always break, but they would break even more if it weren't for chemistry.
OK, but certainly cooling speed is really important.
So let's go to the-- OK, now how-- OK, if you look at glass, remember I said that it's got order or no order, crystalline, amorphous, crystalline, amorphous.
How do you tell which one you have?
Who can tell me?
You just learned about it.
What do you do to see if something is a crystal and which crystal it is?
You shine x-rays on it.
You go to your x-ray source, and you just shine it on, and you measure where it bounces off.
But see-- so if you did that, so if you look in the circle, they look the same.
They look the same.
That's crystalline.
That's quartz.
This is glass.
Quartz, glass, crystalline, amorphous.
Right, but if you go to the long range order, there isn't any.
So if I shine x-rays, these are two different types of solids.
Think about it like DCC FCC, but they're not.
They're more complicated of quartz or of silicates.
This is what glass would look like.
Look at that.
There's still like a little bit of a peak there, but not really, right, because this is from the short range order, but here's the important part, which is where-- you can still, even though it's not that everything is where you think it is, right, because it in a crystal, you know everything's BCC or FCC.
Every lattice is the same.
You know you can count on it for 10 to the 20 second repetitions.
Here you can't.
You can still control the properties.
You can still control the properties.
So the properties can be highly engineered.
And here's an example.
So these are different types of glass.
This is a glass cup, and this is a glass bottle.
And you might think, it's just glass.
No.
It's not just glass.
It's very complicated mixtures of silica with other things.
Notice, it's got soda.
This is-- like I said, this is not soda, but it comes from soda, so it's called soda, sodium oxide in Na2O.
Lime CaO, magnesia, alumina.
All the glass-- you know, unless you pay a whole lot of money for something made of quartz, almost all the glass you buy has this kind of stuff in it.
In fact, 90% of all glass is called soda glass, because it's got-- that's this one here.
That's this cup, because it's got sodium oxide in it.
And the question is why.
Why do we put these things in glass?
Why did we used to put lead in glass?
In fact, the history is pretty-- goes back thousands of years.
And I found this.
If you go to ancient Rome, they made glass, and you can look at what they made glass out of.
They had a little bit less silica.
But they mixed all sorts of things in.
They did not have 3091 back then, so they didn't really know why this was doing something useful, but it was.
Now we mix all these oxides, remember I wrote some of them up here, lime, soda.
We mix those in, and we mix all sorts of other things in, but now we know why.
And the reason why is all about the chemistry, and it all goes back to this picture right here.
It all goes back to exactly this Lewis structure that I started with, which is not on the-- stop it.
No.
It's stuck here.
Maybe it's here.
Yes.
That made me happy.
This all goes back to the chemistry.
If I deliver, if I deliver into this system something that gives me charged oxygens, something that provides a little bit of O minus or O2 minus, then I could cut this bond back.
I can cut this all the way back to the original silicate, sort of.
If I had an oxygen with charge on it, I can go from here back to where I started, right there, at least for that bond, right?
And so what every single one of these things has in common is that it can provide into the system a charged oxygen, a charged oxygen atom.
If this dissociates, it gives we Ca2 plus and O2 minus.
If this dissociates, it gives me two Na pluses and an O2 minus.
Oh, a pattern is forming here, O2 minus, O2 minus, O2 minus all the way down.
So these modifiers, these things that we use to engineer glass, they give me the O2 minus, and that is the knife that I use to cut this glass apart and modify its properties, and it's where we're going to pick up on Wednesday.
But because it's a Friday, I want to throw t-shirts out.
We'll do more next week.
Have a really good holiday weekend.