Flash and JavaScript are required for this feature.
Download the video from Internet Archive.
Description: This lecture explores what it means to engineer glass.
Instructor: Jeffrey C. Grossman
Guest Speaker: Peter B. Houk
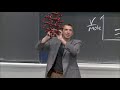
Lecture 26: Engineering Gla...
This is a great day.
We're having our second lecture on glass, and we've got a demo-- where did Peter go?
So we've got a demo coming up for you from the director of the glass blowing lab here at MIT.
And now before we start, I just want to mention, so next Monday-- so we're going to talk today-- we're going to continue what we started, which is the chemistry of glass, and we'll talk about that cooling curve or the effects of different things on the glass formation and then how to engineer the properties of the glass.
Before I start, I just want to mention that next Monday we have something called the Wulff Lecture.
And this is a lecture that is open to the public.
It's geared towards freshmen.
It's put on by the Department of Materials Science and Engineering every year, and it's usually some sort of really interesting, exciting topic and usually a very dynamic lecture.
And in this case it's one of our very own, Sam Shames, who graduated in 2014, and he'll be talking about his adventures as a material scientist and how he's used that knowledge in his life and also in the company that he founded which is now selling products in over 70 countries around the world.
So I think that will be a lot of fun.
If you can make it, it's next Monday from 4:00 to 5:00 PM.
All right, now the second point I want to make is that there's a mistake on tomorrow's quiz, just in the schedule.
So quiz number eight will be given at the beginning of class.
Quiz eight is at beginning of class, not the end.
I think it might mistakenly say the end.
So you might notice sometimes the quiz-- so tomorrow the quiz is going to cover defects and then whatever was covered up until Monday's lecture and Tuesday's recitation, which includes the beginning of what we talked about-- [DINGING]
OK, let's not do that.
Let's turn that off.
And we had an exam on a Monday, and so we didn't want to give you a quiz that week.
So we're doing defects tomorrow even though we covered defects last week.
And then we didn't want to give you a quiz right before or on Thanksgiving.
That didn't seem right.
So for the next quiz what we're doing is we're still making a quiz for you to have, and we're going to give it to you next week but we're not going to count it.
So next week you will still get a quiz that you have for your studying because I know you'll miss it.
But in case you're not here or you don't want to do the quiz over your turkey, we're not going to count that one.
So quiz nine won't be counted.
All right, so that's the quizzes.
Now, where were we?
Here we are.
Speaking of quiz material and thinking about glass as a potential problem on tomorrow's quiz, yes.
You might want to know about some of the things we talked about Monday.
Here's an example.
OK, here's a question.
I have two glasses.
They form from liquids, and they're cooled to some temperature I call T1.
And I tell you that T1 is greater than Tm, which is the melting point of the material.
Now remember, the melting point is the same.
It's a number.
The melting point of silica, that's a number.
But when the material forms a glass can vary.
Why does a glass form?
Well, a glass forms-- this is what we covered on Monday-- it can form because of three different things, right?
One is the crystal complexity.
That's important.
Another is the viscosity.
And the third is the cooling rate.
Those are the three things that we covered on Monday for reasons why a glass might form, and then we started kind of talking about the cooling rate.
That's what we need to understand to answer this question.
So, OK, two glasses forming liquids.
One glass is formed.
Plot the molar volume versus temperature.
All right, so now, let's see.
How about this?
Could this be the volume per mole versus temperature?
So I'm going to try it out here.
I'm going to go like this.
I'm going to go like this, and that looks like the liquid, maybe.
And then I'm going to go like this and maybe this, and we'll call that T1.
Does that look right?
No, this can't be right.
This can't be right because, you see, I said that T1, this is a glass transition.
That's a glass transition.
That's a Tg.
That's another Tg.
But I've said in the question T1 is greater than Tm.
T1 is greater than Tm.
Well, so this can't be true because Tm has to be out here.
Tm is out here where there's a different transition that occurs, and it turns into a crystal.
So this couldn't be right, T1 greater than Tm.
So what about another one?
Let's try another one.
So how about if I-- OK, volume per mole.
Now I'm going to go like this.
So I'm going to go like this.
So now here comes one, and here comes the other.
And OK, so I'm going to put now T1 maybe-- let's try T1 is going to be out here, and Tm would be-- actually, let me do it differently.
Sorry.
I'm going to go like this.
I'll just connect-- how do I want to do this?
I want it to join these up there.
Yeah, that's another good mistake.
I'm making mistakes on purpose.
And let's make T1 be out here.
So T1 is here, and then Tm could still be there.
This could be Tm.
And then maybe is that right?
Is that a possible curve?
Well, that fixed the T1-Tm problem because T1 is now greater than Tm like I said in the question, but what is going on here?
What is going on?
You say, well, but I cooled one faster than the other.
Isn't that kind of what's happening here?
Maybe I started changing the curve?
No.
That's not what this is, so don't make that mistake.
You see, remember, the slope is equal to the thermal expansion coefficient-- thermal expansion coefficient.
And those slopes are the same for the different glasses.
Just because I've cooled one differently, they're not going to have different slopes.
And this is the fundamental challenge.
This is the fundamental confusion point that happens which is that this is not time.
This axis is not time.
So this is wrong to.
What we're doing is we're talking about something involving time, cooling rate.
But we're plotting it on something that doesn't involve time, and that can lead to confusion.
So I wanted to point that out.
You say, well, shouldn't the slope be different because you're cooling it slower or faster?
No, that's not what this slope is.
This slope is a thermal expansion coefficient.
So the right answer to this must be that you have your nice liquid phase and you've made your two glasses.
So here's one.
This has cooled faster.
This is slower.
This would be Tg.
This would be another Tg, maybe Tg1, Tg2.
And the Tm would come down and have-- well, I've run out of room here, but Tm might come down like this.
And so T1 must be somewhere up here.
That would be a correct plot for that question.
So does everybody see?
I just want you to get a feeling for this plot.
We introduced it Monday, and I really want you to feel your oneness with this plot because it's a very important plot to understand why glasses form.
All right, now moving on, there's a lot of properties of glasses, and here I'm talking about oxide glasses, so silica.
Remember, silicon oxide, oxide, silica, alumina.
These are, oh, oxide.
We talked about that.
Chemically inert, electrically insulating, mechanically brittle, optically transparent, visually arresting-- I like that one-- these are the properties that we know about these kinds of glasses.
We already know them.
But what I want to talk about today is how they can be engineered.
And this plot is a basis for understanding that.
And so is spaghetti, and that's what we're going to talk about.
So the very first one-- which we'll set up our demo in just a few minutes.
The very first one that I want to talk about is the mechanical properties.
We know glass to be very brittle, but could we change its mechanical properties?
Could we do something about the mechanical properties?
And by the way, if those are the things that dictate whether a glass forms or not, then those are the things that you might think we might try to engineer if we want to change the properties of glass.
You can imagine I'm a silica-- oh, you got to pass this out.
I'm a big silicate, an SiO4, and I'm connected to these other silicate groups through this shared bridged oxygen. And I'm a bulky thing, and I'm trying to move around in the liquid, crystal complexity.
I'm cooling down, and I'm trying to move around.
And [INAUDIBLE] told me to go that way, but it's actually over there, or there's no building there and I don't know where to go.
Where is the lattice site?
It's complicated.
Glass could form.
Glass could form.
Or maybe I'm a silicate group and I'm like, hey, could you please get out of my way?
You're really slowing things down, you other silicate group that I'm attached to.
Viscous, get out of my way.
No, I can't, viscous.
Or maybe you're a silicate group and you're cooling down and you're like, that was very sudden.
I didn't even realize that happened.
All of a sudden I'm supposed to be a solid?
This is what's happening.
And so instead of getting into this beautiful quartz crystal it doesn't get there.
It doesn't get there.
A supercooled liquid wants to be a solid.
It wants to, but it can't for some reason, for some reason that has to do with those.
So let's just have this in our hands while we're listening and thinking about things, and let me just tell you about this one way-- if I take a piece of glass-- if I take a piece of glass, and now imagine that I take some part of it, like this part here.
And in this part I'm going to cool faster, and in this part here I'm going to cool slower.
So imagine it's a slab of glass, and it's like the edges are cooled at a different rate.
That's actually exactly why a car window, if you look at it through polarized glasses, has spots on it.
Those spots, some of you may have seen these.
Those spots are changes, purposeful changes in the mechanical properties of the glass to keep you safe.
Why?
If you call it slower, then you might have some volume that you want.
The volume here, let's call this Vb and let's call this Va.
That goes along with the graph we used before, b and a.
So it wants V-- what did I do-- b.
This wants Va.
But look at what happens.
If I purposely cool the top really fast or maybe I make spots that I cool fast, then what happens?
Well, this wants to have volume a inside.
This wants to have volume-- well, this cools first.
So this cools first.
So inside, so what happens?
As I cool it now, I have this.
This is now a glass.
This is now a solid.
And I should say glass solid, so it's solidified.
And this wants to have a lower volume-- lower volume.
It wants a lower volume, but that's been frozen at a higher volume.
Why?
I cooled it faster.
That's it.
I cooled it faster, and now the inside is like, wait a second, I'm not cooling so fast.
I don't need such a high volume.
Can I please get a smaller volume out of this?
And the top is like, well, I'm already stuck.
I'm already a solid.
And what happens is it pulls.
And so what you get is wants lower volume pulls on the surface, and that means that this is going to be the top, if I'll just draw it one more time, will be under compression-- under compression, and the inside will be under tensile stress-- under tensile stress.
You can kind of imagine this, right?
You can see it.
I've frozen the top because of cooling rate.
It's right here.
I just cooled the top faster.
The inside isn't cold yet.
It starts to cool, and it tries to solidify at a different volume.
It pulls it in.
But see, that changes the mechanical properties of the surface.
That's called tempering.
That's called tempered glass.
This is tempered glass, and there is no better example of temporal glass than the Prince Rupert's drop, which is the demo that Peter has come.
Here's Peter.
There's Peter blowing some glass.
This is what he does in his free time.
He just throws these big glass-bowling parties.
He also happens to be the director of the glass lab, like I said.
He also teaches the classes, the glass-blowing classes.
There's one for freshmen during IAP, and there's another one in the spring.
They're very popular.
But because you're in 3091, he'll get you in.
He doesn't like it when I say that because it's not true.
[LAUGHTER]
But come talk to him.
He's right here, and I think you've got a demo for us.
So I'm going to let Peter take the stage and show you the best example of tempered glass you can do.
And if you want to use the mic-- if you want to talk.
--a little kit out And one year Professor Cima, when he was teaching this class, told the class that they had to come to the lottery for the glassblowing class, and it's a big class.
And so dutifully everyone came to the lottery, and we already have about 150 students plus 3091.
And the lottery was in room 6120, so we overflowed it by a lot.
So I told Michael, don't ever do that again, please.
Which is why I do it every year.
But we like 3091 students.
We'll try to give them preference.
[LAUGHTER]
Trying to work it for you.
He says that.
How many of you have seen Prince Rupert drops before?
Some people, yeah.
There's a lot of online stuff about them, but you get to see a live one today.
So what's the difference between a Prince Rupert drop and tempered glass like the type [INAUDIBLE]??
Anybody want to take a guess at that?
They're both under stress, but the stress is generated in a different way.
So the dots that were on that windshield are formed by air coolant.
That's how tempered glass is made.
It goes through what's called a [? lear ?] or a rolling conveyor, and it's heated up very, very quickly, and then it's cooled very quickly.
And it's cooled by this array of compressed air jets on both sides that take it from almost the transition temperature-- it just about wants to move but doesn't quite, down to room temperature in three minutes or so.
And that super-rapid cooling is what generates that stress on the-- the difference between the inside and the outside temperature in the glass.
And so it's air cooled, but Prince Rupert drop is cooled by-- anyone want to guess?
Water.
Yeah, by water.
So water is a better coolant, right?
So as a result, you get a lot more stress embodied with the glass, a lot more stress.
And so that's relevant because you don't want to make a window that breaks with that kind of energy and so you get explosive energy all over the place.
So tempered glass is engineered to fail.
It's very strong, but it's engineered to fail so that when it breaks, it breaks into small pieces so that if you're walking along the sidewalk and a skyscraper blows out a window, you don't get huge shards of glass falling down and hurting people.
You get little pellets gently raining down, which isn't such a bad thing.
[LAUGHTER]
So I've got two kinds of Prince Rupert drops that I made here and a vessel in which to demonstrate here.
So first I'm going to show you-- they both cool rather quickly, but one was cooled in air.
One was cooled in water.
This is the one that's cooled in air.
And by the way, I used to give a demo in the Glass Lab where I would take the Prince Rupert drop, put it on a sealed table that we call the marver, and hit it with a hammer as hard as I could.
I was never able to shatter this head with a hammer.
In fact, I dented the marver so many times that I stopped doing that demo.
So I have a different way of doing it now which is-- I won't do that today, but usually I'll have a student come and try to break the Prince Rupert drop in their hands.
It's really hard to do.
Most people can't do it.
But the air-cooled Prince Rupert drop doesn't have the same kind of strength.
So watch what happens.
Not much.
Well, let's try down farther.
Maybe there's more stress there.
Nope, not [INAUDIBLE].
Let me try one more time.
Maybe where it's thicker maybe there will be more stress.
Maybe a little.
So, here's the one that's water cooled.
OK, don't blink.
Five, four, three, two, one.
That's the speed of sound in glass, which is, I think, about three times faster than the speed of sound in air.
So you can't see it.
But there is some great high-speed video-- maybe some of you have seen that online-- of Prince Rupert drops exploding.
And actually up in the Edgerton Center they have a camera that can catch that, and they've got some really cool video.
I'm going to leave this.
This is a very cool fragment that shows the fracture, the failure pattern, which is really interesting.
It fails from the center of the drop radially out at an angle that looks to be about a 45-degree angle.
You can probably explain that.
That will be on the quiz tomorrow.
[LAUGHTER]
45 degree [INAUDIBLE]?
Was there a question?
I'm going to do one more just in case anybody blinked, and then I'm going to be on my way.
By the way, Jeff mentioned that we had an IAP pass that starts during IAP for freshmen and a spring class that's open to everybody at the institute.
So if you're not a freshman, you can come to the lottery.
The lottery is for all of our classes, are shown on the Glass Lab website.
And I think we're going to be doing the lottery for the spring for the IAP class in November late this month, and then the spring class will probably be sometime in January, and it starts in March.
OK, ready?
Five, four, three, two, one.
[INAUDIBLE] will even have some more energy.
Thanks, Peter.
[APPLAUSE]
That was awesome, and I actually do have a high-speed video of it, so I'll show that to you now.
And again, so if you have questions about glass, if you want to watch-- Peter can make animals for you, I think, on request.
Oh, this guy.
But anyway, thanks a lot, Peter, for coming by.
As you know, I'm a big believer in hands on, seeing things.
Can we pass-- is this-- no.
Well, your call.
Just don't eat it.
So now you know on parent's day, when we have parents here, we had the two sheets of glass.
One is called annealed.
It just means it's not tempered, and the baseball was supposed to break it.
It didn't.
Peter's got very good annealed glass.
He's the one that gave us those big sheets.
And it breaks into these huge, dangerous shards.
Thanks, Peter.
Take care.
Oh yeah, thank you.
[APPLAUSE]
If you notice, the tempered glass, we tried to break it.
It also doesn't break.
It was supposed to be the only one that didn't break.
But then you take a little hammer at the edge and you just lightly tap the edge and the whole thing breaks.
Why?
Because it's got so much built-in stress.
It's got all this compressive stress, and the edge isn't protecting that.
The edge of that tempered glass isn't protecting the stress.
So when I just dig in a little bit, it's able to all crack and release that stress.
So that's what happened on family day.
Here's a high-speed video.
These are two guys from Corning.
And we can listen to this.
[VIDEO PLAYBACK]
They're actually pretty cool.
- So there's a few things going on here.
The cold water rapidly cools the exterior surface of the glass, hardening it almost immediately.
The interior, still molten, cools more slowly.
As it cools, it contracts and attempts to pull the surface in with it, but it can't-- well, not very much.
The surface has already hardened, so it gets pulled in only a little, compressing it, while also creating an internal layer that remains forever under tension.
It is this action that gives the glass its uncharacteristic strength.
We call it compressive strength.
- It sounds like the same principle as how an arch provides strength in structural engineering.
- Yes, kind of.
Now, Jamie, I'm going to ask for your help.
We're going to attempt to destroy this Prince Rupert drop.
I just want you to tip that hammer past its center point.
Go ahead.
That's what Peter was talking about.
So you can hit it really hard.
- Now I feel like we've been swindled.
- Swindled not.
We have just experienced-- That's good writing.
That's good writing.
- --the power of compressive strength.
It does, however, have an Achilles heel.
Take those nippers right there and nip the backside of the tail of this Prince Rupert drop and watch what happens.
Wait, wait, wait, cue the high-speed camera.
OK, here we go.
- Oh!
Here it comes.
- That was even cooler than I thought it would be.
[END PLAYBACK]
So that's what you just saw live.
And what that is is it's a relief of-- thank you, or not.
[APPLAUSE]
Yeah, sure.
As Peter said, tempered glass like in your car, it's done with air cooling.
That's literally what those circles are.
If you cool it even faster-- bam, faster cooling.
You now know, what does faster cooling mean?
Well, if it's going to form a glass you might be all the way near the melting point, and you're going to have an even-higher volume per mole which is going to cause even more stress within the glass which is going to make the surface very strong because it's all compressed, but it's also going to have a lot of energy in it that it wants to release.
Let's see.
We were here on Monday, and because I was talking about other ways to change the properties-- in fact, remember, I showed you a couple of different examples of glass, right, a glass cup and a glass bottle, and it turns out there is not just silica in it.
There's all these other things in it, and there's many, many kinds of glass.
And I listed a few here.
This was from Monday, so I'm showing it again.
And going all the way back to glass made in ancient Rome you'll find that it's got these other ingredients.
Why?
So that's what I want to talk about next.
Well, let's use this.
So if you notice-- and this is where we left off Monday-- if you notice something like calcium oxide, well, it's going to go to Ca2+ and O2-.
Let's look at Na2O.
Na2O is going to go 2Na+ plus O2-.
How about alumina?
That's on there, Al2O3.
Well, that's going to go to 2Al3+ plus 3O2-.
Notice a pattern.
The pattern is you get O2-.
We can understand why O2- minus has been used, why chemistry has been used to change the properties of glass for millennia.
And the reason goes back to the pictures that we drew on Monday where we started by talking about the chemistry of the silicate group.
So remember Si?
Remember this?
An SiO4 is happy if it's got four minus charges.
Remember that?
We started with Lewis and it satisfies the octet.
But then in glass what happens is you bridge.
And so you have this.
And I won't draw all of them.
I'll just draw it the way we drew it before.
And you have these bridges, and all of these oxygens are bridges in glass.
So now I'll say, well, what if I wanted-- and remember, these are forming these long, viscous chains.
What if I wanted to make them flow a little more easily?
What if I wanted them to form shorter chains?
What if I wanted to cut the pasta?
Don't do it in Italy.
They'll get really upset.
But Americans are OK with it.
If you need to cut the pasta, it's OK in this country.
But if I need to cut the pasta in chemistry and it's glass that I'm cutting, O2- is my knife.
It's my chemical scissors.
Chemical scissors, I like that-- chemical scissors.
Why?
Well, you can see because I go back.
What did the oxygen do?
By bridging it didn't need that extra electron.
But if I bring now something like Na2O-- so I've got an O2-.
Now I'm going to add an O2-.
Then what will happen is you're going to cut this.
So now I'll just draw the-- I'll see if I can-- OK, so now I've added not just an oxygen atom but the two charges that each of them needed before they bridged, you see, to be happy?
And so now this can have one of those charges, and over here you can have another one, and so on and so on.
But see, now I've cut.
I've cut the glass pasta, and I've done it chemically and I've done it from understanding of octet and Lewis, from basic chemistry principles that we've learned.
Where's the sodium go?
Well, they'll be like a sodium.
They'll want to hang out close by these.
There will be these ions in there.
These sodium ions will be in there still, right?
If it was Na2O, if it's something else and it's a different kind of ion that might be leftover, but the main thing is I've delivered the chemistry needed to cut this.
And this is called chain scission.
There's a term for it, chain scission, the technical term for cutting glass pasta.
Well, this is powerful.
This is very powerful because if I can cut this-- those are the silicate groups, right?
And if I can cut them, then I can change another property.
Look, I can change the viscosity.
I've hit another one of those three reasons why glass forms.
And so I'll show you a slide in a minute that shows you the change in the viscosity, but just to give you a sense of what can happen, how does that impact?
How does that impact?
Well, for silica crystal, the melting point is greater than 1,200 C. For Tg of soda-lime glass, it's 500 C.
So you can imagine now I've got another mechanism.
I've got another way, not just processing, not just cooling rate, but chemistry to mess with this curve.
Because you can imagine now that I might be able to get to lower and lower transition points.
You see this?
I might be able to get to lower and lower transition points if these things can move faster and not get locked into a solid as easily if I add more and more of these chemical scissors.
So maybe this one, now instead of being cooled slower, maybe this one had more soda than this one.
Maybe now they're cooled at the same rate but I've changed the amount of impurities, the amount of oxygen, O2- very specifically, that I've put in.
So it's another way now to change this curve.
Now, these are called-- the thing that forms the glass-- and we're really only talking about silica as our glass.
You can make other types of glass not made from silica.
We're talking about silica in this class.
So silicon is the atom that is making those long spaghetti strands, and it's called the network former.
Silicon is called the network former.
And then the thing that I put in there-- the thing that I put in there that modified the network is called the network modifier.
So you can see on this graph that what you've got is your bridging, the bridging there that's going between two network formers.
And then you've got these atoms that are hanging out near a place where you've broken a bridge.
So those would be modifiers.
Those are called network modifiers.
These are just terms that you will hear and see when you think about glass-- network formers, network modifiers.
The chemistry that you introduce is modifying the network, and it's what is done in many, many different ways with many, many different [INAUDIBLE]
to change the properties of glass.
And so here's the curve that shows you another kind of important way of looking at this.
And I don't need you to remember all of these terms, but I want to show you because this is how glass is engineered.
Remember, today the topic is how do we change the properties?
We talked about the cooling rate.
Now we're talking about adding chemicals.
And so now you're going to work with the glass to do something with it.
If you're going to do that, this is one of the first plots you'll look up.
And it shows you-- it plots the temperature.
This really doesn't work at all-- the temperature on one axis-- so that's like the x-axis-- and on the y-axis is the viscosity because now that's the property that we're changing, right, the viscosity?
So there's silica up on the upper right.
Can you see that?
So fused silica, that's basically almost quartz.
That's basically quartz.
So you are really close to making a nice crystal, but notice how high of viscosity you have versus temperature.
Notice that you're at this very, very high value for viscosity.
It's a really thick material.
And people care a lot about viscosity when they work with glass.
Why?
Because you need to know, for example, is the viscosity at a point where I can work with it?
Can I shape it?
That's the bottom.
That's the region that says working range.
I can still shape it.
I can make a window.
There it flows under its own weight.
So now I can shape it and it will lose the shape.
That's kind of important to know if I'm making a glass animal.
There its stress can still be relieved.
Remaining stress can still be relieved, and up there you can rapidly cool it without introducing new defects.
But look at the tradeoff between the temperature that you have to go to and the viscosity and the viscosity that you have.
So if you go now from this almost quartz all the way down-- OK, there you take a little bit of stuff and you put it in, but it's still 96% silica.
And now you go to boiled silicate.
Remember, it's like 75%, 80% silica plus other additives.
And look at how much you've changed the temperature.
So I can now work with this material below 1,000 C. That has huge consequences for industry, for actually making different things out of glass, which we now do regularly.
And the Romans did it too, but they didn't know why.
They said, well, if you add a little bit of this dirt, something different happens.
Let's try a little bit of that one.
Now we know.
We know why.
And so this curve now is something that we understand and we understand how to control it.
And if you go back to the example of making glass tough, I was just showing you these ions, these sodium ions.
They're left in there.
Well, it turns out that you can put ions into glass, and maybe you're doing it to change the viscosity so the thing stays liquid for longer, and you can maybe work with it in different regimes.
But maybe you're putting the ions in there to do something else.
And so actually there's a whole another thing you can do once you have ions in the glass like sodium.
You can substitute them out.
Imagine that I have-- that's what this next picture is showing.
Imagine that I've got a glass in here, and those red circles are sodium.
But now I've introduced a different ion on the surface.
That's potassium.
What happens?
Well, if I have a high concentration of potassium and I put it on a surface of glass that has a bunch of sodium in it, so now I've got a glass with sodium ions in it.
And I expose it to a potassium bath.
So now I've got potassium up here.
And what happens is if the concentration is high enough and the conditions are right, I can get potassium to go in for the sodium.
And so actually now I can substitute.
So these were here.
I can now substitute those for potassium.
Well, but this is a big deal because potassium is bigger than sodium.
And so if I am able to on the surface, let's say, put something bigger in there, then you can imagine that it's pushing the other stuff into each other.
That's causing the same stress.
K+ plus bigger than sodium, and this causes this huge surface tension.
Same principle as tempering, but now I've done it chemically.
This is Gorilla Glass.
This is all phone screens.
This is what they do because you can get really, really hard glass this way.
And if you go back to these guys, they have a little-- and this is just a very short video.
They've got this sort of longer videos on the Corning website that talk about this, and they shoot bullets through Gorilla Glass.
You can make glass really strong with just chemical substitutions, and all you're thinking about is atom size.
Because by putting this larger atom in, you cause this same kind of compressive stress at the surface.
[VIDEO PLAYBACK]
- Gorilla Glass, it's been refined over time, but like all Gorilla Glass variants that came before it, it is compressive-strength glass.
But it's not made in the same way as we just demonstrated, the rapid-cooling method.
No, instead Corning uses an ion-exchange process.
To break it down simply, the surface ion particles that naturally form during the manufacture are replaced with larger ion particles.
Once exchanged, the larger ion particles create the same sort of inward pressure that we see on the Prince Rupert drop.
And with this method they are able to control and manage the resulting-- [END PLAYBACK]
The resulting what?
What was he going to say?
I don't know actually.
I don't remember.
I have to go watch it now.
So that's just a video showing you what I just showed you, which is that that's yet another way of engineering the properties of glass, and there are so many more.
One of the points I want to make today about how to engineer glass-- so we're talking about how to change this curve.
Maybe we change the viscosity by adding O2 which changes the curve here.
Maybe we change the cooling rate.
Maybe I can use additives, ions in different ways.
But it turns out that we have come to a place where we can engineer every single one of these properties.
We can make conducting glass, even though it's usually been and usually is insulating.
We can make chemically active glass.
As you just saw, we can change the mechanical properties.
There are now flexible rolls of glass that look literally like Saran wrap almost, but they're glass.
And so it all comes down to the pasta.
And so where we are, if we use the pasta analogy-- which as you, I think, can understand I really like because I really like pasta.
This is a great analogy for glass because it's like in the Roman time, all we had was one sauce, everything.
Just mix it all in-- meatballs, pesto, red sauce, carbonara.
I can go on.
And that's all they did.
They just didn't know why things were happening.
But now that we know about the electronic structure of atoms, we can make glass into any delicious pasta dish we want, right?
OK, don't eat it, but you know what I mean.
And so I'll give you a couple of examples.
So the point is the last 20 centuries, we were really just getting warmed up when it comes to this material.
What the recent understanding of how to engineer this material has led to are real breakthrough ideas.
And so I'll show you just a couple in my why this matters.
Because glass is, after all, made of-- there it is up there in case you can't see it.
This is the abundance of elements versus atomic number, and you notice the very top two elements are silicon and oxygen. And so it would be really nice if we could make a lot of stuff out of these really abundant cheap elements.
Think sand.
Could I take sand and make a lot of stuff out of it?
Well, not if I can't control.
Not if I have to heat it up to 3,000 degrees or its viscosity isn't what I want or it doesn't give me the properties I want, but that has changed.
That has fundamentally changed.
So we can now look at materials like this, these super-abundant materials, and we can completely rethink them.
And I'll give you one example.
It's already a few years old, but I think it's just a really cool idea, which is called the Solar Sinter.
And here he's developed this machine that is entirely solar powered.
They're solar cells for the electricity.
And it's a focused beam of light from the sun that gets hot enough to engineer the glass.
So he takes sand from the desert that he's in.
He puts it in a container, and he's got a 3D printer that he's made.
It's entirely solar powered.
There's no fossil fuels, but he can take sand and turn it into something structural.
And so there's a vase that he's made, and you can take that out.
And again, that's just the beginning of rethinking what we could do with these super-abundant materials.
Here's an example from also a few years ago from an MIT lab.
This is Neri Oxman's lab, and she's in the Mediated Matter lab here at MIT, and she's developed a 3D printer.
[VIDEO PLAYBACK]
[MUSIC PLAYBACK]
And so there it is printing something with glass.
And she can print lots of different designs now using glass.
She has a cool video.
And there is the printhead.
And again, 1,900 Fahrenheit.
I'm not sure that I want a 1,900 Fahrenheit printer on my desktop.
But as you can imagine, what they had to do-- I'll just give you one more example and then we'll stop.
What they had to do is understand everything we've just talked about.
How do you engineer the viscosity, the melting point to make it all work in a 3D printer?
The last point I'll give you is this.
I love this.
This is from a few years ago.
It's a group in Japan that made a glass that is as strong as steel.
And what they talk about-- this is what I like.
They say just think of a world where your smartphone wouldn't shatter.
OK, cool.
Buildings could be bolstered against natural disasters, even cooler.
And then somehow they bring it down.
Wine glasses are reassuringly safe.
[LAUGHTER]
Was that really a problem?
I don't know.
And what they did, fabrication was conducted using an aerodynamic levitation furnace where ingredients were floated in the air using oxygen gas and melted together using CO2 lasers, and they get a transparent superglass with 50% alumina.
That was so hard to do because the aluminum didn't want to be a glass.
It wanted to go and become a metal, a crystal.
But by doing it this way, they were able to capture it in the disorder.
All right, have a great night.
See you guys on Friday.