Flash and JavaScript are required for this feature.
Download the video from Internet Archive.
Description: Discussion of energy storage, electrical storage, and the chemistry of batteries.
Instructor: Jeffrey C. Grossman
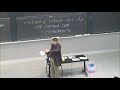
Bonus Lecture 2: The Chemis...
[SQUEAKING]
[RUSTLING]
[CLICKING]
JEFFREY C. GROSSMAN: I want to start with this sort of big picture, all right. So we're going to kind of introduce energy storage, and then we'll talk about sort of why electrical storage is kind of a thing and important, and then we'll kind of go into batteries and then talk about the chemistry of batteries and throw in a couple of, why this matters.
So if you think about this on a planetary scale, actually, the planet is kind of a storage device. It's an energy storage device for that thing over there, right, and so like if you think about all the energy flows that go on on planet Earth, well, then you've got-- there's a picture I like. It kind of shows you the different energy flows, right. You've got the sun, so you've got like solar radiation. You've got tides, there's the moon, you've got wind.
You've got all these kinds of energy happening on the planet, all the way down to life happening, rock formation, right. And then sometimes those things, you know, rocks can form alloys over millions and millions of years. And the thing is, if you think about this, where you draw the line is somewhat arbitrary in a sense, right.
It depends on our use. I mean, if you think about it, fossil fuels are actually a renewable source of energy on geological scales. The problem is that it's like 10, 20, 30 million year old sunlight, right, and it'll happen again, but the question is how do we store energy on timescales that matter to us.
And so you can draw this line and then think about what that means, and typically, most sort of energy storage needs are going to be around a year or less, right.
OK, so now why electricity? Why electricity, right. So the thing is, electricity is more and more important in our lives, and if you look at just sort of like the major sectors, so you have the power generation, you have transportation, you have industry, you have buildings, and this is a plot of these sectors and the CO2 emissions. You can plot all sorts of things about these sectors. This is CO2 versus time.
These are kind of the major contributors, but the thing is electricity, because of things that are happening as well, the opportunity-- either the use of electricity now like power to your home, or the opportunity to use it a lot more later, like transportation, that means that electricity has sort of about a 70% role, about a 70% opportunity of all of this.
That's how much electricity matters here, but the thing is that electricity, as you know, in an electric car it's not going to be useful if you have to have it plugged in all the time, right. And so most of this 70%, most of this 70%, I want to emphasize, is not currently-- it's the potential for the electrification of our world that is happening. That's how much electricity will play a role, but most of it is useless unless you can store it, so storage of electricity is clearly really, really important.
And so we get to sort of how do you think about storage technologies, and again, I just want to kind of gently introduce us to energy storage and we'll go into batteries. Now so the kind of plot that I'm showing you here, talking about Ashby plots, right, but this is actually a different kind. It's a special kind because it has to do with energy storage, and there's a guy who in the 1960s, David Ragone, he first--
And it's actually fascinating. He wrote a paper in 1968, a review of batteries for electric vehicles in 1968, right. And he's saying, how do I put-- there's all these possibilities. We want to electrify our transportation fleet, but which batteries make the most sense? And so he decided to plot them on these two axes, energy stored versus the power and that's a very common way.
These are called Ragone plots now, so it's the energy versus power. And this is how you'll see a lot of discussions. If you look online and you're interested in energy storage as a field, you'll see a lot of plots like this where you got, OK, so energy is like watt hours, right. For example, this would be like watts times hours and this would be like the watts.
So one of the nice things about plots like this, mostly, it's always on a log scale so you can stretch out and see all the different technologies on one plot, right. And one of the nice things about a plot is that this is energy, this is energy, and this is energy per time, right. And so that's energy per time times time, so we can have lots of fun with that.
But so what that means if you think about it a little bit, if you plot energy storage technologies on plots like this, then these diagonal lines are one number of time. It's like if you had a given density and you're using it at a given rate, a giving power, then that's how long it would last. So these plots are really convenient. They're really convenient ways to compare energy technologies.
OK, so those would be like, I've got energy storage technology and it's got this much density, this much power-- well, then it's going to last if I use it at that power for 41 days and so forth. Now the interesting, though, there's lots of stuff on here. Why don't I just want high and high? That sounds like a good thing, right, so shouldn't we be pumping water up hills all over the place?
Well, obviously, if you think about it, you don't do that in your phone. You don't even do that in your backyard. You don't even do that in a town. Why? Because pumped hydro is extraordinarily limited. You have to do it at very large scales. It's very low energy density, right. It's geographically highly constrained.
It is one of the oldest ways we have of storing energy, right. I'm just thinking of the waterwheel, but it's very constrained, so we can't use this in most applications, pumped hydro. But OK, oh, so this is compressed air. Compressed Air, CAES. That sounds good. That's got a whole lot of limitations as well. We've got, just to give you the abbreviations, Superconducting Magnetic Coils.
Superconducters, right, just keep it going. And then you draw the [MUMBLES] and it just keeps going forever. It's a great battery, right, except for all the problems that it has and how much it costs, but it's a really cool concept and it's here. All of these things exist. All of these things exist. They all exist and they're all very interesting and important energy storage devices.
They're interesting, important, electrical energy storage devices, right. So that's the main point. So like here, you know supercaps, you build up a lot of charge in a solid. Here you've got flywheels. Well, I'm just rotating something heavy really, really fast. I get worried about that. I don't want to be in that building, but they do build flywheel energy storage device where you slow it down and then you generate electricity from that.
So these kinds of plots are really, really, really helpful for comparing one energy technology with another. Now, the thing is as I said, there's a whole list of these and it goes on and on. Oh, that's really hard to see. They've all got a lot of limitations, all right, and so capacitors have a low density. You can convert energy into some fuel and store it and then combust it.
That's got low round-trip efficiency. This is what we're going to talk about today, electrochemical. There are challenges there too. Kinetic, pumped hydro, there's so many different ways of storing energy. The thing is there is an advantage that I want to mention about using electrochemistry. There is an advantage about using electrochemistry, and what is electro-- I just said the word electrochemistry.
I should tell you what that is, right, and it's the relationship between electricity and chemical reactions. I love saving time, rxns. That's equal to electrochemistry. This isn't it. Now electrochemistry, OK. And so what you'll see is like things like electrochemical energy storage, and this isn't just one type of thing. It's not just one type of battery. It's not even just batteries.
It's this broader definition, but we're going to talk about batteries today. Now, so there's something else that's really useful. So I mentioned we're getting to the point where we're electrifying everything. We need electricity almost everywhere, even in your combustion car. You need electricity a lot more than you used to, than 20 years ago.
And so as we electrify our world, and then I said I've got to store that energy, you start to think about what Carnot did. Now I'm paraphrasing here, but this is basically what he said if you think about how it applies to this conversion, which is that the second law of thermodynamics makes you pay a penalty of energy if you convert it into heat and then back into electricity.
Well, even if you just have heat in the first place and now you want to generate electricity from that, you're limited. We talked about this before, right, like that the energy density of gasoline, it's so high and it's such an amazing energy storage material because you can transport it safely, right.
Yeah, but the thing is, I just gave you the example the car. You put it in your car. Does anybody know how efficient, how much of that energy your car actually uses to move percentage wise? Take a guess? Yeah, it's 10% to 20%, maybe, 10% to 20%. It depends on the car-- my car probably 5%. It's an old car.
So most of that gasoline's energy is going into waste. Why? Carnot. Now this is not a thermodynamics class, but this comes into this so I wanted to mention Carnot and the fact that there is another way to do it. So you could do chemical reaction to heat. That's how we power most of our world today.
A power plant gets up to a higher temperature so the penalty is less, but still most power plants, you lose about 50%. Most power plants at best are around 50% efficient in terms of converting the thermal energy into electrical energy. Yeah, but I can use a different way, and that's electrochemistry. And it's one of the reasons why electrochemical energy storage is so appealing, because you don't really pay a penalty.
You don't really pay a penalty. I can go back and forth without the second law hurting me. So I want to mention something about Carnot, about the second law, and again, this is not a class on thermo, but the second law involves entropy so I had to say something, especially because it's in Averill, right, and that's your textbook that you're diligently reading every day, and there it is.
And by the way, Averill has a beautiful chapter on batteries, chapter 19. I'm sure you know. It's really well written. This is what he says about entropy, though, and for now we can state that entropy is a thermodynamic property of all substances that is proportional to their degree of disorder. Averill, such a good book, but let me ask you guys a question.
So people think about entropy as disorder and they think about entropy as sort of more disorder. Let me ask you, which one of these has a higher entropy, the one on the left or the one on the right? So how many of you think the one on the left has a higher entropy? How many of you think it's the one on the right?
So the thing is it's the one on the left. Yeah, right. I love that you guys are blown away. Why? Because entropy is not about smoothness and it's not about disorder. That is wrong. Entropy is about accessibility to states. It's about how many states you have to be in. Now, that can appear to be like disorder, right, but a messier room does not have higher entropy, and in this case, it's just simply the rules of the algorithm.
I have rules here about the dots being able to touch each other. They are randomly selected but with the constraint that they cannot touch each other. That means that those dots had fewer possibilities. They had fewer states that they could be in. This system has much higher entropy. It's about the number of states. I just want to make that-- again, this is not the topic of this class, but I've got to make my case about entropy when I can.
And I brought up Carnot, so there you go. Entropy is not just disorder. Now OK, so we're back to batteries. Now so electrochemistry, there's a lot of reasons why electrochemical storage is-- here's the chart again, right. It's this one here. You see that, electrochemical batteries, all right, that we're going to talk about.
There's a lot of reasons why this is so appealing. No penalty on the second law is one of them. You can have transport easily, right. We drive electric cars. We see that. No pollution at the point of use-- pollution can be focused at the point of production. Also, the batteries can be charged by renewable technologies like solar wind, and they're very highly efficient.
Electric cars, for example, have a very high efficiency depending on the car, but upwards of 90% in terms of converting that electrical energy back and forth. The metrics that matter, I wrote a couple of them here, all right, watts, hours. These are the things that matter in batteries, but so many other things matter, and this is why this is a complicated problem.
And it's also why batteries are not in any way a one-size-fits-all. I mean, you know that from like AAs and AAAs and 9-volt, but it's so much more than that, because it's about the application change not going from your computer to your phone but going from your car to your phone to a house to a grid.
And so the needs are all going to be different, and then which ones of these things you care about is going to depend on what chemistry you think about. It all comes down to the chemistry as we're going to see. It's all about the chemistry. And just to put a few numbers down, the cell phone has a few watts of power. Light bulb is 10 to 100. A AAA battery has 1.5 watt hours of storage, all right, so that's how much energy is inside of a AAA battery.
Needed to drive a car 200 miles, 100 kilowatt hours. It's just fun to think about these things, right. Powering your house, 40 kilowatts max, depending on how many AC units you're running. The world is this many watts as of a few years ago. So if you had, I think it's something like 20,000 billion AAA batteries, you could run the world for an hour-ish, something like that.
These numbers are just fun to play with, so I wanted to put a few examples down. So where'd it all begin? It all began with an argument. It all began with this beautiful argument that I have to tell you because it's really a cool piece of history. And it took place in Italy. And it was Galvani who was a physiologist, and he really loved the topic of why animals move, right.
So what is motion? How is it that animals are moving? You know, how am I moving my muscles? How is anything moving? Well, there had been theories about this going all the way back. You remember Socrates and Plato, the elements, right, and aether? There were similar theories for motion of the human body. In fact, the word pneuma was used to describe the essence that goes through all living things that allows them to move.
Well, Galvani didn't like that and he wanted something more concrete, and so he was really trying to make a connection, especially between things that move and electricity. Now, it was difficult. There wasn't like a constant source. So you had electric generators in your lab at the time, but Galvani wanted to go big, so he hooked up his lightning rod to a frog.
The frog was not alive. Well, the frog was not alive, seriously, because then you'll see why-- at least I don't think so. I really hope not. Now I'm thinking about it, I'm getting a little bummed honestly, but I don't think they were alive. That doesn't look alive. So he hooked them up to lightning rods and he watched and he waited as the storm's coming, and he's like, the lightning rod hits and he hooked him up to some metals, and the frog went crazy.
It was like, ah-ha, motion, electricity. He'd been jolting them in his lab with his own electric generator, but he wanted to go big, and also, they didn't know. They didn't know at the time what electricity was, right, so they didn't know if the electricity that he generated in his own thing was the same as the electricity that came from the sky. He saw that you get the same result. The frog still moved.
So Galvani said the motion of a frog, and all motion of all living creatures, generates electricity. That's what he said. He deduced that the motion itself is something that generates electricity. This is a really cool little article about this debate, because in Galvani's case, if you have two metal wires hooked up to a frog, like we say, if you close the loop, then what he said is that the charge that was already in there moves around the loop and back and makes the leg move.
Volta got really interested in this and he said, you know, let me study this. He was a physicist at the time and he said, this looks fascinating, let me study it-- but he said, you know, why do you keep using two different metals? So I think this is about the metals, not the frog, and they had a big huge argument, right.
So this is Volta's picture, is that it's when you connect two different metals that you get a charge flowing through the frog. Volta, I think, as many of you know, went on to win that argument, and we have the volt named after him, and he also created the first stable battery, the voltaic pile. Galvani on the other hand really opened the doors to the idea that we have electricity in our bodies, which is pretty cool.
He also is the reason why the story of Frankenstein was written. It's actually true because they went around and took all sorts of things and electrocuted them and showed that they moved, and often they weren't alive, and so Mary Shelley, I think, saw that. You went around like the countryside shocking people, and I actually meant that as like not literally, but just shocking them by what he's doing.
And I don't think they ever used humans, but still, people's imaginations and so forth, and it was quite a thing. OK, very interesting story. Now, why does this matter? I've got two why this matters. Today my first one is on a personal scale, smaller scale, and then my next one is on a grid scale. So this is called a little sun.
There's over a billion people who can't read at night because there's no access to electricity, and of course, a lot of the studying that you could do would be at night, right. So this is a really cool program. Why does this work? This is a solar cell but this wouldn't work unless you could store the electricity, right, so I wanted to make you guys aware of Little Sun.
You just press this and you can read at night, right. This is already making a big difference in millions of people's lives. That doesn't happen unless you've got electrochemical energy storage, right, and there's some stats up here. $25 billion liters of kerosene are used to meet the basic lighting needs in a lot of these places. That releases tremendous amounts of toxic fumes, and you can make a huge difference, But you know, it's sort of the same as cooking, right.
So wood fire cooking is a huge problem. So the challenge is, but you don't cook. If you have a solar cooker, that's great except that most people don't cook during the day. They cook at night or in the morning, right. And so you've got to store energy to make these things actually useful, right, and I really like this program so I wanted to make that one of my, why this matters.
And we'll go big on the next one, but let's get to the chemistry. So what happens in a battery? The best way to understand this is with a classic example of two different metals. It's Volta's two different metals. Volta did lots of metals. That's what he did. And he showed that the frog moved with most of them, so it's the metals.
So let's take these two classic metals and show what happens. You've got copper and you've got zinc. Now, in this case, I'm just going to have a zinc. This is the zinc piece of metal, and this is a piece of zinc, and I put it in a solution of copper ions. And so in this case, what do we have? Let's write this down.
In this case, I've got zinc solid. Well, before I write this down, let's write down what's in the solution. The solution is copper sulfate and this goes to everything that we've been talking about. So I've got a solution, a beaker, that has copper ions in it. How much? I don't know. Look up the K sp, right? Now we know how to do that.
So 2 minus in solution. So you put some of this copper sulfate powder in that beaker. It turns blue and it gives you these ions that are dissolved in solution, and then you put a piece of zinc in there. So what happens is there is a transfer of electrons, right.
Now we talked a lot about electrons. We're talking about transfers of electrons. I mean, just think about it, right. We went from H, right, like this. Look at that, right, the bonding and then we did this, OK. Here we go. It's a little bit less sharing but still. All right, and then we did this, where it's like, OK, there's almost nothing there.
It all went there. These are where my electrons are, all right. Well, then we put this in water and we got this, OK. And we got them sort of separated and this one just broke off with its electron, leaving a positive charge behind. So we've done all this. We know this, all right.
So what's happening here is special because what's happening here is a trading of electrons. You see, in all the bonding that we've done, we're talking about how electrons share in bonds and then these things break apart, but now I'm going to do reactions that trade them. That's electrochemistry. That's electrochemistry, and what happens when I put that zinc strip into that solution is this.
The zinc that goes into solution is 2 plus and 2 electrons. And the copper in the solution plates onto the zinc, plus those 2 electrons goes to copper like that. And if you look at the full reaction, the full reaction would be then zinc solid plus copper 2 plus in solution goes to zinc 2 plus in solution plus copper solid.
That's the trading. Now I like writing it like this because you really see explicitly the gaining and losing of electrons. Oh, those have words associated with them, gaining and losing electrons. But this is the reaction that we're kind of used to, right, so this is just the same thing. It's what happens, right. It's what happens. It's a trade of electrons.
Now it turns out that when something loses electrons like that we call it oxidation, and when something gains electrons we call this reduction. And so when two different materials-- in this case, these two metals-- trade electrons and one of them is oxidized and one of them is reduced, that's also got a name. It's called a redox couple. Those are a redox couple, redox, redox, right.
So these are a redox couple, is what they're called when you trade electrons like this. OK, so what's happening? What's happening is at the surface of the zinc, I see these copper ions in solution. And it's like, well, if I gave you two electrons, then you'd be able to become a copper solid, right.
So if I give you two electrons, you're a copper solid, but now if I lose two electrons as a zinc atom, I'm a zinc 2 plus. That's this. That's just this, right. Why does that happen? Well, we'll get to that, right, but that's what's happening. So a zinc atom at the surface, all right, a zinc atom at the service sees a copper 2 plus and it says here, have 2 electrons.
Oh, and then it's like, wait a second. I gave two electrons away. I've got to go into solution. And that's exactly what you see over here, right. The copper is plating the zinc and the zinc is coming off of the strip. They're doing this. All right, why is that happening? Because if you put that strip in there and the thing just heats up, this is exothermic.
That means that this reaction will go. This is spontaneous. This is spontaneous. Where can I write that? Nowhere. Spontaneous. It didn't have to be, as we'll see, but this one is. But all that's happening is you're going down in energy. You're giving energy away and you're heating up the environment.
Now the question is, how can I not let that happen? How can I stop the heating from happen and instead take advantage of this electron trading to do work? That's what a battery is. That's what a battery is. It's taking advantage of this. And really what it comes down to is just a different construction of the device. It's this. The
Fundamentals of a battery is this kind of thing, but now instead of allowing this plating to happen, I'm going to separate that and I'm going to construct it in such a way that those electrons get traded through some wire that I can do work with, OK.
So for example-- where is it? OK, maybe not for example. Maybe for example, maybe I need to turn this on and off. Here we go. This is the picture that Averill gives for the battery. So what you saw wasn't a battery because I wasn't stopping the electron flow and doing anything, but if you construct it like this, is called a galvanic cell.
All right, if you construct it like this, now I can do work with that electron trade. I can do work with the electron trade. That's the difference. So let's go through this. So on the left, so what did I do? Well, I got my zinc strip but now I've got a copper strip. Just trying to see. OK, I've got a copper strip over here. I've got a zinc strip over here.
But I'm putting the zinc not into the copper directly where I just short it and the whole thing just heats up. Instead, I'm putting the zinc into a solution of zinc ions. Look at that. Zinc has zinc ions in solution, right. Now, copper, copper is in a solution of copper ions. So these are now the two beakers.
On the left I've got zinc nitrate. In this case it's a nitrate, not a sulfate. It doesn't matter. The whole point of the solution is that I get these ions in a beaker. Got to get these ions in a beaker, so you've got the copper ions in a beaker on the right with the copper solid and you've got the zinc ions. So let's look at what's happening and blow up those different sides, right.
So at the anode, it's called the anode because it's where the charge comes from. It's called the anode. What's happening? Well, what's happening is what I just showed you. The zinc is leaving the solid. The zinc is leaving the solid and it's becoming 2 plus. It's becoming 2 plus. But the thing is that now, you've got to be careful here.
So the zinc is becoming 2 plus. What about those two electrons? Where do they go? A zinc atom leaves its metal strip and goes into that beaker of zinc ions, right. But now you've got two charges, two electrons on the strip because it left a zinc 2 plus. Nothing more will happen. Nothing more will happen unless those 2 electrons go away.
That's the whole point. What's so cool about batteries is that it's all about neutrality. If you think about batteries as current flowing, batteries is all about neutrality. It's all about keeping these things neutral because if I just build this charge up here, I can't have another zinc atom go in so the charge has to go somewhere, but that's what that wire is for. That's what this wire is for, right.
This wire allows the charge to go over to the other place, the cathode, where the copper is sitting there saying, you know what, if I had two electrons-- if I had two electrons on the copper strip then I could use those as a way to lure in a copper 2 plus ion, right. I could use those as a way to lure it.
And if I did that, then I'm doing what this did right here. It's the same thing that we just did with the plating except now those electrons are going through this wire up here. The zinc is trading electrons with the copper. That's keeping this neutral, so now the electrons go away and now another zinc can go into solution.
Then the copper grabs them, plates a piece of copper, and then more electrons can come, right. It's the same thing. It's the same thing. This is what it looks like. If you take those electrodes out of the solutions after running this for five, 10 minutes, look at what happened. The copper plated.
You got copper ions from solution plating that and zinc ions from solution leaving that. And so those are the reactions that are happening. One's happening on the zinc side and one's happening on the other side, but there's something else. There's something else because there's just one other piece of it that you've got to understand to understand how a battery works, and it's this thing here.
So up here, this is my conduit for electrons to go. Each time it goes, I can plate. I can lose the more it goes, right. But notice if I lose 2 plus-- so I've got these 2 plus. It's not charged. Look at this. Copper sulfate in that case is copper nitrate. Copper sulfate goes in a solution, it's not a charged solution. These things are balanced, right, 2 plus, 2 minus.
So now I'm taking only a 2 plus out of solution. No way. It's the same thing. You've got make it neutral again, all right. It won't work otherwise. You can't start building up charge in the solution. So that's what this thing is about. That's what this is. This is called a salt bridge and that completes the circuit. See, this lets the electrons flow. This gives ions. It doesn't matter what it is, right.
That's the electrolyte. It's just a source of ions. Here is an ACL. It's an ACL, so now for every copper 2 plus that plates onto the copper electrode, two NA plus atoms go into this beaker from the bridge, because here I've got dissolved salt. Dissolved salt, sodium chloride is in water, so I got all these sodium ions. I got all these chlorine ions.
And when a charge imbalance happens because something plates here, I can draw sodium atoms out of the salt bridge and I can draw chlorine atoms out of that side, right. That completes your circuit. That's an iron neutralizer, the salt bridge, and this is allowing the electrons to keep everything neutral, and that's how you get the current, right.
It's pretty cool. It's pretty cool stuff. Now the thing is, you can do this yourself. That's why I bought these for, is that you can-- somebody sees the the potato battery. I like the human battery. So these are just volt meters and these are set to the right thing, yep. And what you'll find, you can see what happens, right.
Like when you touch the two electrodes, yeah, and sometimes you've got to squeeze. Oh, look at that current. You have electric-- you are providing electricity. You are the salt bridge. You are the bridge, so what that means is you are actually plating and taking metals off of these two electrodes right now. Your hands are doing that and you're trading electrons. You're a conduit for that to happen, right. You're the salt bridge.
It's pretty cool, and you can play with these. You know, you have volt meters. You have lots of items on the table tomorrow maybe at a dinner, and I'm just suggesting that you play around with this idea, because look. This is what Volta did. This is literally what the name of that device, the guy that that's named after, this is what he did.
He hooked up two different metals. I just put some paper clips in there, it works. It can be almost-- not almost, but lots of different metals will work. Why? We'll get to that in a sec. Here I've got another paperclip and a copper wire, right, two different metals. The power isn't coming from you as Galvani thought. No, the power is coming from the difference in potentials of those metals wanting or not wanting electrons.
Everybody wants electrons, let's be honest, but who wants its more? It all comes back to the same stuff we talked about. It's just that now we're talking about the metals and whether they're more interested than the other metal in having those two electrons. That's what a potential is. That's what a potential is.
Oh, now so OK. Here we go. There's the volt. So what determines it, right? What determines the potential? What determines the potential? Well, it's what I just said. You know, intuitively, it's the difference in these things wanting and saying, well, OK, I got copper and zinc. How do I know? Well, if you look at zinc-- let me see. Where do I have it? Here we go. Zinc, I want to make sure I get this right.
So zinc is argon and 3d10 and 4s2. All right, OK, copper, oh, we got numbers 30 and 29. Copper is the same thing in terms of its argon core, 3d10 4s1. Which one of those wants to give up 2 electrons or take 2 electrons more than the other? That's what determines the potential.
And you know, when you look at this, you might say-- this is from Averill so he actually is missing the f's because this is just taken right from the textbook, but anyway, so that's the potential. Look at this. This is like an energy diagram but these aren't electronics. These are just potentials, but the potential is related to which one wants the 2 electrons more.
It's 2 plus. The oxidation state is 2 plus, so you look at this, you say, well, I don't know which one might give up electrons more. It turns out it's the zinc. And you might say, well, but wait a second. If I just take an electron out of here, won't it be harder than one out of here, because this only has 4s1?
The answer is yes, but I'm taking 2. I'm not taking 1. I'm talking about the oxidation state of 2 plus. That's going to be harder here. It's harder to take a 4s1 in a 3d from that beautifully filled 3d shell than it is to take these 2s electrons. That's why that's higher. That's why that's hire, right.
So what you can see is that the potential difference is all about chemistry, right. The delta v is all about the chemistry. It's all about all of the things that we've learned. It's more than that because of these solutions, so it's more complicated than that, but at the core of all of this is all the topics that we've learned, how strongly are electrons bound to an atom, to a metal, right.
And so well, if I took cobalt, what would happen? If I took cobalt instead of copper, the voltage here would be 1/2. And you can sort of look at the table and think about why that is. Cobalt has a 4s2 but it's got less d electrons, and so is it going to be easier or harder to pull them out? Well, it's going to be easier than copper but harder than zinc so it goes in the middle, right.
And so the potential difference is less for cobalt and zinc but you still get a potential. And so that's what Volta did, and he did all sorts of different metals.
And you can understand why zinc and copper does this but copper and zinc doesn't. That's copper in the zinc solution. That's zinc in the copper solution. Nothing happens. The reason is the potential. It's just this. It's just literally the potential difference that we just drew. This is going to be easier to take.
So I'm not going to go the other way, right. I'm not going to go the other way, so nothing happens when you do that. And then finally, the last kind of piece of this is that we need a reference because you can only get the changes in potentials. It's like enthalpy. You can only get the changes, not the absolute, so you've got to have a standardization.
And the standardization in the world of batteries is hydrogen. So what you do when you think about battery potentials is you write down all of these reduction-- reduction is gaining electrons, right-- you write down all. It doesn't matter. You could write reduction, you could write oxidation. They're just a flip of the sign, right.
It's just a flipped sign of the potential, but what you write these down with respect to a common electrode, and it's the standard. It's called the standard hydrogen electrode. So sometimes you'll see it as the SHE in the battery world. And what it is it's just a very nice platinum electrode that doesn't change. So it doesn't plate or lose atoms. And it's a reaction that on that electrode happens with hydrogen, hydrogen gas.
And so what you do is you hook this up on one side. You hook that up on one side, and then you put all the metals against that. And you measure their potential.
What's so powerful about the SHE is that now I've got all of these with respect to the same reference, right? So now when I look at my zinc-copper battery, well, it's just the difference between those that's the potential of the battery. So hydrogen electrodes gives you that ability to standardize it and create huge tables.
And if you look up potentials of reduction, they're all related to hydrogen. You get thousands of them. How much does somebody want to lose, how many electrons? That's going to be in these tables. And it's by a setup that is very similar to the one I showed you that is related to hydrogen. OK.
Or you could go the other way. If I had zinc-- I just showed you zinc. Copper in zinc doesn't happen because it's the wrong sign. But then what if I put copper-- instead of in zinc, I put copper in silver. So there's copper in zinc, copper in silver. It works. Because now silver is down here.
How did I know that? Because I hooked it up to hydrogen and I compared it to copper. And so that's how you do battery chemistry is you look things up. You look up these potentials in tables, and you think about which voltage you need.
And that gets us to this Nobel Prize. You say, well, why are lithium-- why did the Nobel Prize go to the-- not discovery but the development of lithium ions as a storage technology? And the reason is this. You saw-- it was the highest one on the list, too. Look at that. So now, I don't know what the potential of a lithium something battery is going to be, but I'm starting out with a pretty negative voltage with respect to hydrogen. That makes you think you might get high voltages, and you do.
Yeah, but you have another thing that's really cool about lithium is how light it is. And those things combined make it a beautiful battery technology. And that's why the Nobel Prize was given. Because if you look at it on a plot like this, it's all the way up here, if you look at energy density versus volume.
And a lithium battery works much the same way in the sense that you have the separator here. That's the electrolyte. But now it's rechargeable. And I don't have time to go into rechargeable batteries versus what are called primary batteries, which is the one I showed you. But all the chemistry is the same. You're shuttling ions back and forth. You've got that external circuit.
And what I wanted to-- and there's a whole bunch of chemistry. So what has exploded in the last-- well, that's a bad word for batteries because that is a danger with lithium batteries.
[LAUGHTER]
But what has really made the field take off is you know the word. We're in the class. It's chemistry. Because the flexibility of where you can put that other electrode and what properties it has gives you enormous room. That's why lithium-ion batteries can go in everything now, all the way up to the grid. And that was my last Why This Matters in the last 2 and 1/2 minutes.
This is the grid now. So we went from small to very big. The growth in solar and wind has been incredible over the last 10, 20 years. The problem, as I think I've shown you, is the variability. And so this is like-- if you zoom in here, that's the load, the black line. And this is what these two resources give you. And you can see that it doesn't match. If you zoom in on a day, then you really can see it, right? There is the load. And there's what you would get from solar and wind.
And so here's the question. I said, well, OK, how do you fix this? You got to store it. There's no way to use renewables at large scales unless you store it. In fact, we're really at essentially the tipping point. Because if I look at the countries in the world and what their SPV penetration is-- and this is a couple years old, but it's still pretty much the same-- Germany, Greece, Italy at the top. Sad frowny face here for the US of A.
But Germany is at 7.1% as of a few years ago. That's a huge penetration of PV. Well, here's the problem. If you look at-- this is Germany, now. Huge penetration-- these are these six years, seven years of adding PV. This is how much they had. They're now at 7%. 2016 is 7%.
This is how much the price of electricity was in Germany when they added. So notice that here is the sunlight electricity during the day. They can sell it for a lot of money. But as they add more and more of this renewable to the grid, its price goes down when it's available. In fact, it's now not economical for them to add more PV unless they can add it with storage. Because actually the price has gone down so much and it's gone up here when they're not generating any PV electricity. So you can come up with-- and they did in this paper-- this sort of value factor which, when it goes below 1, means there's no economic outcome that's positive here. You can't make money any more.
And look at where that happens. It's right around where they are. It's at 5%. You can't solve renewables at the scale of the grid without storage. And no option exists today. It might be batteries. It might be batteries, but there's nothing that actually does it today at the scales that we need. So that's a real challenge for chemistry.
And I hope you guys have a great Thanksgiving and maybe hook up some different metals. And I'll see you all next week.