Flash and JavaScript are required for this feature.
Download the video from iTunes U or the Internet Archive.
Topics covered: Electrons in Chemistry: Redox Processes
Instructors/speakers: Prof. Christopher Cummins
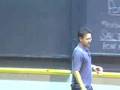
Lecture 22: Electrons in Ch...
Related Resources
The following content is provided by MIT OpenCourseWare under a Creative Commons license.
Additional information about our license and MIT OpenCourseWare in general is available at ocw.mit.edu.
Onto today's topic.
Today we are going to be focusing on issues associated with oxidation-reduction half-reactions.
And I want you think back to my first lecture, where I really talked a lot about how unifying the concepts were that stemmed from the ideas of Gilbert Newton Lewis, because we talked about acid-base, donor-acceptor, electrophile-neucleophile, and oxidant-reductant all as parallel concepts, with the related theme there being what is going on with the electrons in the system.
And it is really important to consider not simply the reactions that occur between molecules, but also the reactions that occur when electrons coming from molecules or coming from ions or coming from metals are connected up in some kind of external circuit that can be interfaced then to some real world problems through the mechanism of electrochemistry. And so we will talk today about some of the basic concepts that you will need.
This will be the equipment that you can use to do future research to solve the world's energy problems.
This is going to be good. And it is pretty straightforward. I want to give you an example of a reaction that can be decomposed into half-reactions.
And that is the reaction of magnesium metal with carbon dioxide. And this will be two equivalence of magnesium going to two magnesium O two plus elemental carbon.
Now, this actually is an important reaction in that it contains one molecule carbon dioxide, that is really a critical energy and environment molecule that we worry a lot about today. I will emphasize this in a few points during my lecture today, but we want to be able to find ways of producing energy that don't simultaneously produce a whole lot of CO two that goes up into the atmosphere and is a greenhouse gas. And so we will talk about that.
I would like you to just start thinking in the background of your mind right now about the molecules, if you can list them, that are really important to energy concerns today.
With this reaction, we can decompose this into the following half-reactions. We can see that it can be written as carbon dioxide plus four electrons plus four protons going to carbon plus two H two O.
And then simultaneously we can also write two magnesium plus two H two O going to magnesium two equivalence O plus four H plus plus four electrons.
Although the reaction that one may actually carry out would be the one on top here, that reaction can be separated out into the parts that are associated with oxidation and the parts that are associated with reduction.
And so, down here in equation one, we see that CO two is undergoing reduction by four electrons.
This is a four electron process.
And down here, in equation two, what we are seeing is that this magnesium metal is ultimately serving as a source of four electrons in that process, each magnesium going to magnesium two plus.
There is implied, here, that we are going to be able to know something also about oxidation states.
And so, as we talk about oxidation-reduction processes, I want you to also, if necessary, review about what you know about the assignment of oxidation states in systems. But by writing this out, you can see that on the left side of equation one, electrons are going in. And on the right side of equation two electrons are coming out.
And, really, if you can keep track of what the supply of the electrons are and where the demand is for the electrons in the overall system, you will really have a good feeling for how these processes physically may be taking place.
And then, up here on top, this is the sum of those two equations, one plus two. And that is typical of how we write oxidation-reduction half-reactions.
And when we consider a pair of half-reactions, one of the things that we are really interested in is the potential difference.
And that is to say if we have some way, physically, of sequestering the two individual half-reactions into different containers, and if we allow them to communicate, but we don't allow the reaction to proceed to any significant extent, we can measure this.
We can measure it when the reaction is not allowed to proceed to a significant extent.
And the reason for that latter caveat is that when you put into communication two sequestered cells that are each poised, one to undergo a reduction and one to undergo an oxidation, then if you put them into contact and let that reaction start to proceed, you can see that that reaction would proceed until such time as equilibrium is reached.
And so all throughout that process, the potential difference between the two would be changing until it stops changing. And when it stops changing, we would have reached equilibrium.
The two independent half-reactions at the beginning have their own potential, the degree to which they are poised to undergo oxidation or reduction.
And then, how do we do this? Well, you are going to see that we can use a thing called a salt bridge.
And with a salt bridge, we can allow for not only communication of the electrons from one of the half-reaction cells to the other, but we are also going to need to allow for the movement of ions.
This permits ion movement.
And this requirement for ion movement stems from the necessity to maintain electroneutrality, charge neutrality in each of the two cells that we have, each of the two compartments of the electrochemical cell that we are going to create. And in reference to electrochemical cells, -- -- there are lots of different types of electrochemical cells.
And today we are going to be discussing two of these in particular. We are going to start out by talking about what is called a Galvanic cell.
And then, I am going to talk about how you can use Galvanic cells to measure standard properties of oxidation-reduction half-reactions.
And then, after we do that, we are going to talk about the properties of redox-active systems.
And then, finally, I will finish up by talking about another type of electrochemical cell, and this will be an electrolytic cell.
So, let's look at a Galvanic cell.
Last week I left my blue chalk in here.
Gone. Oh, well.
Down one color. If anyone can find that blue chalk for me, I will be very appreciative.
As I have been alluding to, we can set up an electrochemical cell. In this case, called the Galvanic cell, in which we sequestered two different half-reactions to the different compartments.
And then we are going need for a control of the communication between the two compartments. And we will do that in the following way. What I am representing, here, is a piece of metallic zinc.
And the way I have drawn it, it is supposed to be like a simple strip of zinc metal, to which we could attach some alligator clips and can run an external piece of wire over to the other side. And we can interpose, here, some kind of a meter. This round unit, here, could be either a volt meter or an ammeter to measure, respectively, voltage or current, in a system like this. And then, over on the other side, we will have a different electrode.
And this electrode will be made of metallic copper.
And I have got to have my salt bridge so that we can maintain electroneutrality in these solutions that we are going to have on both sides in the two separate compartments.
So, there is my salt bridge. And this might contain an electrolyte, such as potassium nitrate.
And that electrolyte would be suspended in a gelatinous medium like agar, for example. And what we are going to be interested in will be the directionality of the electron flow in a system like this. We are going to be interested in the potential that gets set up when the electrons flow in a system like this. And we are going to want to know which side is the anode and which side is the cathode, and what are the equations for the reactions that are taking place at the side that is the anode and at the side that is the cathode. Let's look at that.
Let me just point out that if I had blue, I would be indicating the aqueous solution here in blue.
Maybe those of you who are colorblind will think that is blue, but anyway. The idea is that we have an aqueous solution here, on both sides.
And this one, over on the right, is going to start off with some concentration of copper two ions -- -- in solution. So, some molarity of copper two will be present in the solution over here on the right-hand side, where we have the copper electrode. Inside the salt bridge, we have potassium cations and nitrate anions that can go into solution on either side to balance the charged changes that are taking place as oxidation-reduction reactions happen on the left-hand side and on the right-hand side of this electrochemical cell, which is a Galvanic cell.
And so, what we find is that you let this go into contact briefly. And you would measure, for this cell, 1.1 volts.
And so, that is the magnitude of the potential difference between the two half-reactions that are present on both sides of the cell. And, furthermore, we would see that the electrons are starting out over here, and they are going this way around the circuit.
And that tells us that what we have on the left electrode at zinc is going to be called our anode.
That is because at the anode, -- -- oxidation is taking place because metallic zinc is undergoing oxidation and becoming zinc two plus and providing, in so doing, two electrons for every zinc that gets oxidized.
What you can think of as happening over here on the side that we call the anode is that you have this piece of zinc metal. And, in order for electrons to start coming out to the external circuit, for every two electrons that comes around to the outside of the circuit, you have a single zinc two plus ion that jumps into solution. A piece of zinc jumps off of the surface of the electrode. That zinc atom leaves as the zinc two plus ion. That supplies two electrons to the external circuit. And, at the same time, to balance charge, we would have to have, from the salt bridge entering solution, two NO three minus, two nitrate anions, because they are singly charged, have to come out of the salt bridge and into solution every time a zinc two plus drops away from the electrode and starts flowing into the external circuit. And electrons go around the initial voltage when the reaction has not proceeded to any significant extent, is this 1.1 volts.
So, we have measured the potential difference between the two half-reactions. Here is one of these half-reactions. At the other side, if on the left side we have an electrode that is the anode, then on the right-hand side we must have an electrode that is the cathode.
And we must have reduction occurring there.
And what is getting reduced? Well, as electrons appear down here at this electrode, they can encounter a copper two plus ion in solution that can then become part of the surface of the electrode as a copper metal atom.
In other words, we are depleting the surface of this piece of metallic zinc over here.
And zinc ions are going into solution.
And, on the right-hand side, copper ions are coming from solution and becoming incorporated into the electrode itself. And so, if you ran this reaction for a while, and then you took the two pieces of metal, zinc and copper, that you had used to set up the Galvanic cell, you could weigh them. And you could see that the zinc electrode would have gotten lighter, and the copper electrode would have gotten heavier.
And so, that is also the basis for the technique of electroplating, where you can take one metal and cover over the surface of it with a thin layer of another metal. Here we are just putting a new layer of copper on the surface of this copper electrode, and we are depleting what we call, over on the left, the sacrificial zinc anode. And this reaction then, over on the right-hand side, is copper two plus plus two electrons going to copper metal.
So, that is a prototypical example of a Galvanic cell.
And in setting that up and thinking about what is happening there, in terms of depletion of one electrode so that the other one can be increased in mass, you may be aware that you know of places where this principle is actually used.
In construction, for example, there are large steel bridges that are constructed.
And one does not want those to oxidize away and to become fragile in their structure, so that bridges break down and bridges are not safe anymore, shall we say.
And so, what is often done there is large pieces of zinc, actually, are put into electrical contact with the metal of the bridge so that it is the zinc that actually gets oxidized away as a sacrificial anode, instead of the bridge itself. The same for any of you who are sailors or boaters in general. You probably know that zinc anodes are present usually in several places on boats so that electrolysis does not occur and corrode away the metal parts of your boat. Instead, the zinc sacrificial anode is oxidized away and is a source of electrons.
So, that is an important principle.
Once you have started looking at some different kinds of oxidation-reduction half-reactions, you are going to want to know just how oxidizing is something or just how reducing is something.
And, in order to do this, we need to pick one particular half-reaction that will serve as our universal standard.
And, to that half-reaction, we will compare everything else. And so, that brings us to the discussion of standard reduction potentials, or standard potentials.
For this, I am going to make another Galvanic cell.
And, concerning this Galvanic cell, we will have a number of the same questions that we have had before.
But the one that we are going to be using on the right-hand side is going to be very special.
Here we indicate our water line, again, in green.
And I am going to put in a salt bridge into the system.
And I will have an electrode, here, on the left and another electrode over here on the right.
We will connect up these electrode using alligator clips.
We will need an external circuit with a meter, so we will be able to compare the potential of the two half-reactions of interest. But then, over here on the right, this electrode is going to be a little different.
Because this one is going to have something like a test-tube inverted over it -- -- because the reaction that we will talk about here, in fact, does involve a gas.
And that gas is hydrogen, another one of our important energy molecules to be talking about in the context of today's lecture. At the end, I would like to see if you have a list of energy molecules collected from today's lecture. But also, we are going to choose, as the electrode material, here, this metal piece of electrode material.
This is going to be platinum. And the reason we are going to choose platinum, a very common electrode material, is that platinum is a so-called noble metal.
And that means that it is quite impervious to most chemical reactions. Over there, we were talking about zinc and copper electrodes that themselves do change during the reaction. And over here, we want to have a piece of metal that can provide the valuable function of giving us electrical contact of the chemical reactions on the two sides, but an electrode that will remain clean and unchanged at its surface throughout the course of the reaction. So, we use a platinum electrode over here. And you can see that with this inverted test tube through which the wire passes and with some kind of a provision for a side arm attachment here, we can hook up a cylinder of H two gas.
And we can start bubbling H two over this electrode, like this, so that it actually will have bubbles of H two coming out like that. In fact, this solution over here will be saturated with H two.
And a further consideration for our reference electrode, which is going to be known as the standard hydrogen electrode, or SHE.
That is, the electrode against which everything else is going to be compared. And it also has, in the solution here, 1.0 molar H three O plus.
So, indeed, it is a very strongly acidic medium. And what we are measuring, over on the right-hand side, is a half reaction that may correspond either to two H plus plus two electrons going to H two.
And that will be the case if this side is the cathode.
But if this side turns out to be the anode, then we would be measuring the opposite reaction.
And I will talk more about that in a second.
Let's say, for example, that our electrode over here is, in fact, made of zinc.
If our electrode over here is made of zinc.
And we may have zinc two plus in solution, here, and we may again have something like potassium nitrate or some other electrolyte present in our salt bridge. Then what we can do is put these things briefly into contact.
And we want to know two things. We want to know what the magnitude of the potential difference is and what the direction in which the electrons are flowing is so that we know which one is the cathode and which one is the anode.
And, in this particular case, the direction of electron flow is, as before, away from the zinc.
Once again, zinc atoms are jumping off the surface of the electrode as zinc two plus ions.
And, every time that happens, two electrons go into the external circuit and come around here.
And the chemical reaction that occurs here, in this case, is that those two electrons that came from one of the zinc atoms react, as shown here, with two H plus ions to make H two.
And so, we know the direction of electron flow, we know the exact reactions that are taking place on the left and on the right. And we know that, for this particular choice of substances, our anode is on the left, and our cathode is on the right.
And let me come over here.
There is another way that I can write this cell.
And so, I would like to introduce cell notation to you.
And that will be like this. We will have zinc.
And then, a solid line. And then, zinc two plus.
And then, a solid double vertical line.
And then, H plus, another solid vertical line, H two. And then another solid line.
And then platinum.
That is the way I set that up.
We could, actually, add another solid line here and put platinum over here. The thing that I want you to recognize about this notation for Galvanic cells is that the solid single lines represent interfaces, direct contacts between things. Here, it would be a platinum solid electrode connected with solid zinc, possibly. And then, the way I drew it over there, it would just be solid zinc, no platinum on the left. And then this solid line represents a solid liquid interface because the electrode is dipped into a solution that contains zinc two ions. And that is in communication with the other half of this electrochemical cell by a salt bridge. And the salt bridge is represented by the double vertical line.
And then we have, in solution, protons. And then, a solid liquid.
Well, actually, in this case, a liquid gas interface to the gaseous H two.
And H two is also dissolved.
In order for this to be used as a reference electrode, we have to pick standard conditions.
And so, we are usually talking about one atmosphere of hydrogen for the standard hydrogen electrode.
And we are talking about a concentration of 1.0 molar of our strong acid. And so, those are our standard conditions, along with 25 degrees C.
And then this solution that contains the protons and the hydrogen gas is in a solution solid interface with the solid platinum electrode that is not going to be undergoing any change. So, these are the types of cell notations that you will encounter when looking into electrochemistry. And we found out that the left side, where the zinc metal is going to zinc two plus, is our anode.
And also, the potential difference, when we measure it using our voltmeter right here by turning on the contact very briefly, is around 0.763 volts. So, that is the magnitude of our potential difference. And this is equal to delta E zero cell. When I write E zero, that means standard potential. And this is written as a delta here because that is the difference between the potentials for the two half-reactions that we have written up there. But it is a very simple difference precisely because the standard hydrogen electrode is our reference electrode. Over here we want to write the definition of standard cell potential.
And that is a delta E zero for your cell is equal to delta E zero for your cathode minus-- Sorry, this is not delta here.
Just E zero for your cathode minus E zero for your anode.
But E zero for the standard hydrogen electrode, because this is our reference electrode, is equal to zero at all temperatures.
And that is by definition. So, the standard potential for that set of conditions that constitutes our standard hydrogen electrode is taken as the zero of potential for comparison with any other type of half cell that you might be able to consider. And so, we can see further that, in this particular case, where we have our cathode as the standard hydrogen electrode, we have zero and minus E zero for the anode, which we measured as 0 volts.
That means that for the reaction zinc going to zinc two plus plus two electrons, which is our anodic reaction, we have a standard potential of -0.763 volts. And generally, what you are going to be interested in, as you consider different kinds of substances from throughout the periodic table with reference to their ability to take place in oxidation-reduction reactions, is you are going to want to know what your standard potential is. You are not going to always have a system that you want to consider that is under standard conditions. These standard potentials always are referenced to some standard conditions, as I mentioned specifically for the standard hydrogen electrode.
And so, part of next lecture on Monday is we are going to show you how to handle systems that are not under standard conditions because then you can handle some real practical problems. But let's look at a different type of cell.
Let's write down the following cell.
This new cell that I have written the cell notation for, instead of drawing up the whole diagram of this Galvanic cell, happens to be one in which we have platinum electrodes on both sides. Here we have the silver | silver plus redox couple on the left-hand side. And we have the redox couple of H plus with H two on the right-hand side.
So, the right-hand side corresponds to the standard hydrogen electrode, and the left-hand side is a new redox couple, or a new half-reaction, that we want to compare to the standard hydrogen electrode so that we will know, on an absolute scale, where it falls relative to all the other half-reactions we might want to measure. And so, we want to know, which way do the electrons flow?
And, in this case, it turns out that the direction of electron flow is this way. So, this is the opposite of what we talked about in the case of comparing zinc to the standard hydrogen electrode. Electron flow has been reversed. What that means is that this over here, the SHE is now our anode.
And now the silver-silver plus electrode is our cathode. And we want to know not only the direction of electron flow, the direction of electron flow tells us what is doing the reduction and what is doing the oxidation, but we also want to know the magnitude.
And this one turns out to be, this delta E cell, is equal to 0.8 volts. And, by the definition of standard cell potentials that I gave you over there, you can see that what we are getting now, because the electron flow is reversed, our sign is reversed, and so our E zero for the reaction Ag plus plus an electron going to silver is equal to 0.8 volts, positive.
Notice that the zinc-zinc two plus couple was negative with respect to the standard hydrogen electrode, but because the electron flow is reversed for silver plus, silver redox couple, we now have a positive potential relative to the standard hydrogen electrode.
What would like to arrive at is a nice big table where we look at standard potential -- -- with reference to this standard hydrogen electrode, which is our zero of potential. And you will be able to find a table like this in your book. What we found is that zinc is down here at -0.763, so that was zinc-zinc two plus at a negative potential relative to hydrogen plus electrons. We found that up here at +0.8, we have the silver-silver plus redox couple.
These are thermodynamic quantities, so you can look on a table of standard reduction potentials and you can tell which direction electrons will flow if you set up cells that involve those redox half-reactions.
Another electrode that we used today was copper two plus combining with two electrons to give copper metal.
It turns out that one is positive also by about positive 0.3 volts.
You see hydrogen is here and it reduces silver-silver plus.
Hydrogen reduces silver plus to silver because it is up there. Zinc is down here.
Zinc also serves as an anode with respect to any one of those three because all of those three are at a potential positive relative to zinc on this scale of standard reduction potentials. You can also have some other potentials, like way down here at about -2.7 volts is the sodium-sodium plus redox couple, way down there at -2.7 That is why sodium is so much fun to heave into a body of water.
I mean, it is fantastic. You get reduction of the protons to make hydrogen, which then explodes.
So, this is fantastic. And some of you may know that there is an annual, and I am not recommending that you do this, by the way. You can see how negative the potential is down here. Metallic sodium is a very strong reducing agent, indeed.
A very important reaction is up here at about +1.23 volts relative to the standard hydrogen electrode.
And this important reaction is oxygen plus four H pus plus four electrons going to two H two O.
So, this +1.23 volts is very important.
In the time remaining, I am not going to be able to tell you about electrolysis. I believe next hour I will start off by talking about electrolysis.
And, if you can do electrolysis using oxidizing and reducing equivalents that derive from photovoltaic cells, so you are converting sunlight into separated electron whole pairs, you can drive a reaction like this the other way and learn how to make oxygen from water, and ultimately also hydrogen from water. We will talk about that a little bit next time. Have a nice weekend.
Free Downloads
Video
- iTunes U (MP4 - 106MB)
- Internet Archive (MP4 - 186MB)
Audio
- iTunes U (MP3 - 11MB)
Caption
- English-US (SRT)