Flash and JavaScript are required for this feature.
Download the video from iTunes U or the Internet Archive.
Instructor: Dennis Freeman
Description: The final Signals and Systems lecture explains how audio playback evolved from the fragile Edison cylinder phonograph to durable modern optical disks, through the application of digital signal processing concepts.
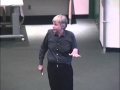
Lecture 25: Audio CD
The following content is provided under a Creative Commons license. Your support will help MIT OpenCourseWare continue to offer high-quality educational resources for free. To make a donation or view additional materials from hundreds of MIT courses, visit MIT OpenCourseWare at ocw.mit.edu.
DENNIS FREEMAN: Hello. So welcome to the last lecture in 003. So there are more lectures scheduled. But I don't believe in trying to cram new material in the last couple of hours before the exam. So no new material after today, although it will be helpful if you continue to try to work on problems, and try to internalize what we did more recently. That's the idea.
So I'm not trying to pump new information in. That doesn't mean that there aren't things that you should be doing. But after today, no new information. In fact, today there is no new information. There will be a session on Tuesday. But the idea is primarily to get feedback from you people.
As I mentioned last time, we've changed a lot of things. We change a lot of things every term. And our primary source of information is the end of term surveys. Tell us what works. Tell us what didn't work. Tell us what you liked. Tell us what you didn't like.
If you didn't like the electronic submission of homework, tell us. If you didn't like the tutor, tell us. If you would rather have individual office hours instead of block office hours, it's a good chance for you to tell us what you think.
And it's especially important that you fill out the online subject evaluation. So please, if you have a laptop with a certificate on it, feel free to bring it to lecture next time. That's one of the main ideas. But we'll also spend some time just discussing what you would suggest for improvements for next time. Questions or comments?
OK, so today is mostly just for fun. Today is mostly just to tell you about the way 6.003 plays out in technologies that you might have some interest in. And I chose a technology that I am particularly interested in. Because as I probably have mentioned in the past, like many of you, I was afflicted with an addiction with music at about your age. I recovered. You will too.
But I was very interested in it. It was a very hot topic. And I want to talk about some of the things that 003 has done to make that industry very different today from what it was when I was an undergraduate. It may not surprise you, but it surprises me that the technology that we used was really not very different from the technology that the inventor invented.
So who invented the phonograph? You can look at the notes. You're supposed to know that. Just shout. Makes me feel better.
AUDIENCE: [INAUDIBLE]
DENNIS FREEMAN: Thank you-- Edison. So Edison was, of course, a genius and a prolific inventor. At the time he invented the phonograph, he was interested in two things that he was trying to draw relations between, telegraphy and telephony.
We talked about telegraphy before. Telegraphy, telegraph, was a way of getting messages point to point. So person A wanted to get a message to person B. And it would be transmitted via the telegraph system. Now I kind of oversimplified the way the telegraph system worked when I talked about it the last time. It really worked a lot more like message passing than you might have thought.
What would happen is that there wouldn't be a direct connection between person A and person B. It might have to go through six relays. So in order to get to California, it might be important that a message that originated in Boston would go to New York, and from New York to Pennsylvania, from Pennsylvania to Chicago, et cetera. So there may not be a direct route over long distances.
So the way that was done, you would take your message to the telegraph office A. The operator would key it in. It would get written down by the receiver, say, in New York, who would listen to the message, write it out, basically reproducing your message there, then key it in to the next relay. So it might actually be keyed in a dozen times before it hits the final destination. And that was tedious, laborious, hard.
And Edison was interested in fixing that. And to do so, he invented a paper tape system, so that the receiver wouldn't need to listen it. They would just punch out paper tape as the clicks came in. So as the dots and dashes came in it, it would make holes in paper. The holes in paper, then, could be used to transmit to the next station. So Edison did that.
He was interested in thinking about whether you could do the same sort of thing with other kinds of signals besides telegraph signals. And that's the origin of the phonograph. He was interested in, could you send a voice message to a relay, have it automatically recorded, so that it could then be rebroadcast over the next link? So he was kind of imagining a telephone network that was based on the same principle that the telegraph network had been.
So the idea was to try to record an audio signal of the type that would come across a telephone wire. So he got together with his aide, Batchelor, and made this drawing. This was 1877. And 30 hours later-- kind of rapid prototyping for the time-- his machinist made this. So that's the first phonograph.
The idea was you wrap that disk with a piece of wax paper. You turn the crank. As you turn the crank, this thing is connected to a screw, so that it's moving this way at the same time this big thing is going around that way. This is a diaphragm, so that you shout in here. And there's a needle connected on the other end, so that as you shout, the needle gets moved with audio frequencies contained in your voice.
So how do you do this, then? So you grab the handle. You put a new sheet of wax paper on it. You grab the handle. You turn at a constant speed, and shout. OK, it took some coordination.
The remarkable thing is it worked. So Edison said, Mary had a little lamb. And on replaying it, the device said, Mary had a little lamb. The very first try, it worked. Now it didn't work all that great. What do you suppose happened?
AUDIENCE: [INAUDIBLE]
DENNIS FREEMAN: Noise? Of course, there was noise, yes.
AUDIENCE: [INAUDIBLE]
DENNIS FREEMAN: Frequency was terrible. Anything more disastrous? Yeah?
AUDIENCE: [INAUDIBLE]
DENNIS FREEMAN: Cranking is not at all constant. So you can make it sound like Alvin the chipmunk if you like. Something worse. How long do you think the recording lasted?
AUDIENCE: [INAUDIBLE].
DENNIS FREEMAN: Yeah, about once.
AUDIENCE: [INAUDIBLE].
DENNIS FREEMAN: So the problem was, to get the energy back out, you have to-- so after recording-- so clean sheet of wax paper, crank, crank, crank, yell, scream, and now reset the needle to the beginning. And now crank without screaming. In order to get the message back out, you had to push on the needle, so that it would follow the indentations on the wax. But if you pushed hard enough for it to follow it, what would happen?
It would erase the message. So it was kind of a destructive read out. It didn't last very long. Because the act of reading it back out tended to erase it. So he redid the thing with tinfoil. That worked much better. And in fact, it caught on like overnight.
So within two years, he was invited to the National Academies of Sciences to give a talk on this. That's him posing at the National Academy of Sciences with his then phonograph. They loved it.
Now despite the fact they loved it, nobody knew what to do with it. Everybody thought it was just the neatest thing in the world. But they didn't have a clue what to do with it. And of course, Edison, one, was a bit self-promoting. So he wrote articles about it, and tried to explain. In the patent, he listed about seven or eight things you could do with this thing. Today they sound a little far-fetched.
Recording important historical addresses, medical transcription-- the one that seems most-- I don't know-- sort of gruesome to me, recording the last will and testament of a person as they're dying. Now that sounds gruesome to me. Then imagine what it was like back then.
Back then, nobody had ever heard somebody's voice who was not alive. The technology didn't exist. So all of the sudden, he's proposing to record the dying breath of, say-- it kind of mortified everybody.
What Edison didn't like was the idea that it would be used for music. That was trivial. He was doing important science. So he didn't like the idea that this would be used for music. And so he downplayed that his entire life.
Nevertheless, the commercial success was music. He was trying to promote this as a system for recording speech, medical transcription, buying breaths, historical talks. That didn't really catch on. This was a playback device.
This really did catch on. This was about 60 years later. It's virtually identical. It became a piece of furniture that everybody had in their house. The cylinders had gone back to wax.
Alexander Graham Bell had worked on the problem. He figured out how to make long-lasting wax. This crank cranked a spring. So you could crank it up, but over time it would play. So you didn't need to go at exactly the right speed anymore-- a number of big improvements. The cylinders held two minutes of recording. So they weren't exactly long-playing.
OK, this was the same model of phonograph I had in college. And it's actually remarkably similar. So there's some differences. There was no crank. We had electricity. It had a motor. So the motor propelled it. It was no longer a cylinder. Now it's a desk. There was no screw. So how does the needle track the grooves if there's no screw?
AUDIENCE: [INAUDIBLE]
DENNIS FREEMAN: There's a spiral. So the records had a spiral. That's right. This was the thing that read the grooves. What held that in the groove? It was gravity. So it was the same kind of idea. You had to push on the needle to hold the needle in the groove, so that the needle could report the vibrations that were recorded there.
But this was much classier than the version in Edison's time. And in fact, you could tell how serious the audio file was by the different kinds of performance enhancements of this sort of thing. So for example, this little knob, this controls the weight, the force that's placed on the needle. If you had a good set up, you could set it for about 1.2 grams. If you had a bad set up, it'd go up to 2 grams.
So you could tell how serious a person was by how much force was required to track. So for example, the primary thing that determined how well the system worked was the cartridge. So here's a blow up of a cartridge. The cartridge-- anybody who is serious had a diamond-tipped needle. If they didn't have a diamond-tipped needle, you just didn't even think about them.
So everybody I knew had a diamond-tipped needle. That was just the way it was. The least expensive kind of cartridges was a little piece of piezoceramic. The piezoceramic was stiff, so that, as you played the records, they tended to wear out. Because you had to put a lot of force on it.
Mine, I had the V15 type 4. So this was a magnetic device. So there were a tiny little electromagnets, so that the up and down motions would be converted into an electrical signal by magnetism, not by piezoelectric effect. And you could track that with a much smaller force.
And I should just mention just so you know that we were serious back then-- so this cost 160 then dollars. There's been a factor of six or so inflation, this cost $1,000. And if you were serious, you had these. So that's kind of the technology as it existed when I was an undergraduate.
And it was good. It had existed for over 100 years. But it was still basically the same thing Edison had done. The media-- oh, I should show it just in case you've never seen a media. So this is a record. This was my record.
And the problem is, of course, they scratch. So over time, these things would scratch. So the technologies for things like, how do you keep them from scratching, how do you wear them out? And you can also appreciate sort of where the music industry is coming from. This costs about $15-- 15 then dollars. So with factor six, it's about $100.
So from the point of view of buying music, it was much more expensive back then. And from the point of view of the recording industry, think of it. You had all these addicted people wanting to buy this stuff. And it wears out. So you play it for two or three years, you have to buy a new one.
OK, so you can sort of appreciate where the music industry is coming from. Because things have changed. OK, so distortions, expensive, fragile, blah, blah, blah.
Then in the 1980s, everything changed. In the 1980s, there was the invention of the CD. The CD was invented by Philips Corp and Sony Corp working together. And just everything was revolutionized.
So we'll talk a little bit about how the CD works. The important thing for the discussion is that, unlike records, CDs are virtually indestructible. They have very low distortion. They are next to free. And all of that is technology that's in large part enabled by 6.003. Of And that's what I want to actually talk about.
So what is a CD? A CD is not very different from a record. So a CD has tracks. The information is written in tracks. The tracks are written as a spiral. The spiral starts at the inside and spirals out. And so the idea is that you read a spiral of messages.
And just like a record-- the way you make a record is called embossing. You make a master. And then you take molten plastic, and press it, and copy the patterns to make that record. In fact, there is a big technology on the formula that you used for the vinyl.
One of the secrets of RCA at the time when I was in graduate school, they had a secret recipe that included dropping four slices of American cheese in their vinyl producing. Because somebody had accidentally once dropped a sandwich. And those records came out good. So there were magical things like that that were trade secrets at the time.
OK, so that technology was basically embossing. And that's the same thing you do when you make a CD. CD is poly carbonate, which is injection molded. So you make a master. The master is usually made out of aluminum. The master is made very similar to the way that Edison would make a master. There's a big device with an electromagnet with a small stylus and it punches them.
But then you copy the CDs by injection molding. So you take that original. And there's actually a complicated process. You use the original to make a half a dozen fathers. The fathers are used to make mothers. Each father makes 20 mothers. Then each of the mothers make children. And so you end up being able to get a lot of copies out of the original master.
There's a reflective layer of aluminum that's evaporated on top of the polycarbonate. And then there's a thin layer of plastic put over the top. That results in something that's extremely robust. And we'll see in a minute how they make it robust.
But this dimension is like over a millimeter. So this is the CD version of the same album. So this surface is the information reading side. There's a millimeter of stuff here. There's a millimeter of polycarbonate protecting this surface that has the information from the environment.
So in fact, if you want to destroy somebody's CD, you might think the way to do that would be to write in felt tip on here. No, don't do it that way. Use a ballpoint pen on this side. Because that layer of plastic is very thin. So use a ballpoint pen here. It will completely screw it up. So it's very robust when it gets imperfections on the read side.
OK, so that's kind of the structure. It's virtually indestructible is the point. So then, how do you get the information on it? Well, the information is coded in these patterns. The patterns are small. The pattern width is half micron.
A human hair is 50 to 100 microns thick. And I have white here. Well, I used to have blonde hair. I have gray here now. Ignoring that for the moment, I used have blond hair. Blonde hair is about 50 microns diameter. Black hair is about 100 microns diameter. So depending on the color of your hair, it would obscure 30 to 60 tracks if you were to lay a hair across it. So these are very, very small patterns.
And so the trick for how you code them is a big problem. Before we get there, though, how do you code the audio? You won't be too surprised to find out that it's a sample data system. So we do sampling just the way we've talked about it before. Audio, you can hear sounds from about 20 hertz to about 20 kilohertz. This is an ideogram.
Audiogram's plot is a function of frequency. What's the minimum amplitude required to be able to hear that frequency? You're most sensitive in the kilohertz region. The scale over here is DB in something we call sound pressure level.
Sound pressure level is the centigrade equivalent for hearing. So 0 is just audible. 100 starts to hurt. 120 is permanent damage kind of thing. So you can hear about 100 decibels over a range from 20 to 20 kilohertz.
And so the way they coded it for the CD was sampled at 44.1 kilohertz. They chose that funny number to be a little bit bigger than twice the highest frequency that you can hear. But they made it the funny number because they wanted it to be an integer multiple of 60 so it was easy to synchronize with television signals. That's where the funny number came from. So 44.1 kilohertz divided by 60 is some integer.
The problem is, of course, that they wanted to be greedy. They wanted all the bits to code audio. So they only sampled barely over the frequency that was required. So according to the sampling theorum, you need 40. So if you had a sample data system running at 44, any amount of signal above 22 would alias and sound terrible. So you need to put an anti-aliasing filter to prevent that.
We've kind of cheated the whole way through 003. We always said, when you need a low-pass filter, use an ideal one. Well, they don't exist. we use ideal filters because they're conceptually simple. There is no such thing.
If you actually try to make a filter using designs like Russ talked about in recitation. Butterworth filters, things like that, they have transitioned bandwidths. So there is a range of frequencies for which the filter is neither fully on nor fully off. And it's in transition. You would like that range of frequencies to be very small, so you don't waste bandwidth.
So they would like to reconstruct up to 40. And they allowed 4 kilohertz in the guard band. That was intentional, because they didn't want to have a lot of bits that were not useful for recording audio. That's the reason they only went up to 44, instead of, say, 80, which would have made the anti-aliasing problem simple.
But it complicated the making of the anti-alias filter. Because there is sounds that come into microphones about 20 kilohertz. It's just that you can't hear them. But if you were to record those sounds, and then sample them at 40 kilohertz, you would hear the alias. And it would be highly objectionable. So you have to anti-alias.
And that's actually hard. Because if you'd like to hear up to 20, and if you're sampling at 44.1, 20 reflected down to 24.1, which means that you want the transition band to be 34 kilohertz. And if you think about the design of a filter like a Butterworth filter, in order to get a transition that attenuates by ADDB-- think about the dynamic range of hearing. You have to make the loudest sounds small enough, so that you can no longer hear them. So in general, you have to attenuate by about ADDB.
You need about 50 poles. To get an ADD attenuation using a Butterworth design in 4 kilohertz at 20 kilohertz. Everybody sort of know what I'm talking about? That's impossible. Lining up filters-- so the way the Butterworth filters work, you remember? So you have a bunch of poles like so.
The way you think about that is that you start with a design that looks like a not-so-good low-pass filter. Then you add these poles, which give you a peaky response like that, so that when this is going down, the peakiness here helps to cancel that. Then you do that again.
So you can think about these successive pole pairs as sharpening the transition by pushing up on the center frequency and rolling off on the edge frequency. But in order for that to work, they have to all be lined up. So if you've got 50 of them, you've got to be able to line these up to about a percent or so. Otherwise, they don't perform the way they're supposed to, and they can actually make things worse.
So making an analog filter of that type was impossible then and is difficult now. Here is sort of the state of the art. So this actually does have ADDB of roll off, because it's a fancier design. As an elliptic design. The trick here, though, is that all the parts are laser trimmed. So they're all done on one substrate. All the inductors, all the capacitors are done on a single substrate.
Then they're laser trimmed, so that they follow the exactly the right places. Then it's encased in aluminum to help to hold the temperature constant and to keep the electromagnetic interference out. And even so, this has just 11 pole pairs. So this is not nearly adequate for that kind of a design.
So what we do instead is we use something called oversampling. So instead of sampling at 44.1, which is what you would think would happen in a CD, you sample it four times that. By sampling at a very high frequency, you make it easy to make an anti-aliasing filter. Because now, you only need to worry about saving the frequencies up to 20 kilohertz. But you've got 170 kilohertz to do the transition.
So the filter design becomes very easy. In fact, a typical design only has five poles, and so just a handful of capacitors. Just two capacitors and two op amps will do that. So then you've got a system that is sampled at 176 kilohertz. What's the problem with that? What's the problem with sampling at 176 kilohertz?
Too much information. So what you've done by sampling at the higher frequency is you've increased the amount of information that needs to be stored by a factor of 4. So instead of being able to record 74 minutes of music, you can record 74 divided by 4, 18 minutes of music. Nobody would like that, right? So how do you fix that?
AUDIENCE: [INAUDIBLE]
DENNIS FREEMAN: Downsample, exactly. So what you do is you run that discrete time signal that was sampled at 176 through a discrete low-pass filter, which you implemented digitally with arbitrary precision as much as you want. So here's a design based on a filter whose length is 200.
By making a length 200 discrete time filter, you can capture the basic sine T over T shape that you're trying to get with an ideal filter. So here with 200 samples, it's easy to reproduce a large number of ripples similar to the large number of ripples that I wanted over here, but now with digital multiplies, not with resistors and capacitors. And this shows where the poles-- this is an all 0 filter. It's a finite length filter. We didn't talk about that.
It's an easy way to implement filter design. It's an easy way to design filters. It's a very popular way of doing it. So it's an all 0 filter. And you can sort of figure out by thinking about the spacing of the zeros relative to the unit circle, how that implements a low-pass filter. That was done with an automated optimizing algorithm for finding the best low-pass filter. The method is called the Parks-McClellan algorithm.
And it makes a very nice filter. So this shows the transition of ADDB passing 20 kilohertz signals and attenuating 24 and above. So you get a very nice filter by doing that in discrete time.
So then having done the digital filter, you can throw away the frequencies that would alias and then downsample. Yeah?
AUDIENCE: Is the filter in those zero locations standardized by anybody? Or does any manufacturer--
DENNIS FREEMAN: Any manufacturer-- yes. Every manufacturer is different. What's happening over time, though, is that the chips are becoming standardized. So if you buy a particular chip, it has a particular filter set. But it's not standardized in the code.
OK, so that's how you generate a signal that is robust. And it's basically just 003. Now we have to think about, how would you make the player? Because what we'd like to do is enable you to use a CD in a more portable fashion than you could use this technology. So the question is now, how would you make a player that can pick up this information off the CD, so that you can reproduce it in a Walkman or in a more portable environment?
So I had this brilliant idea about two years ago that I would take this CD and I would use my microscope. I may have mentioned I light microscopy. I went into my microscope. I used my research-quality microscope, which costs $70,000. And I'll take a beautiful picture of the bits on here. And I'll write a problem set for 003 where you have to decode the bitstream. I thought it was brilliant.
And it was like a week before the homework had to be written, you know, the standard thing. And of course, I couldn't get it to work in time. The problem was that even my $70,000 research microscope has trouble seeing the bits. The bits are really small.
So when I first tried it, I couldn't see anything. So I fiddled around with the microscope. I tried all different kinds of magnifications. I tried all kinds of different optical tricks. But I know I couldn't figure out anything. I couldn't get it to work.
So I got my graduate students. They're always much better at this sort of thing. And we finally got it to work. We cheated like crazy. So we had to use something called water immersion. So you put a drop of water on the surface, so that you can use the-- you lose resolution when you go through a glass to air interface. That's a bunch of microscopy that you don't need to know.
But you do. So there are tricks in microscopy where you can avoid having the air interface by using water. The water is more closely optically matched to glass. So what we did was we did immersion microscopy, put a drop of water on here, put the lens in the water. And that's the picture I got.
So the point is that I had to work to get a $70,000 microscope to read the bits off here. And I sort of gave up with the idea that I would record a track. Because it was just too painful to just get this one image.
Now the trick is they make players that cost $10. What do they know that I don't know? So here I am with a $70,000 research microscope having a difficult time getting the bits off. How do they make a CD player for $10? Just for fun, I did the same thing with a DVD. After I figured out how to do the immersion microscopy, I figured I'd at least-- so this is a DVD done with the same sort of thing.
So how do they do it? They do very clever things. The first trick they use is interference. So I talked about interference last time with our standing wave illumination microscope. They do interference. So I told you that this is polycarbonate with an aluminum coding. The aluminum is actually working like a mirror. And that's the intent.
So they don't actually try to code block and white. What they try to code is distance. So the idea is that you take a laser beam, and you out reflect it off of the CD much like it reflects off a mirror. And then the features that were embossed are at different depths.
And the depth's chosen very specifically to be lambda over 4, the wavelength of the light. They use a 700 nanometer laser. And the features are offset by lambda over 4.
The idea is that-- so if you have a feature, and you have-- so you compare, what would be the timing for this laser beam if it struck the mirror? You compare that to what it would be if it struck a feature. And you rig it so that those two times are different by lambda over 2, lambda over 4 in, and lambda over four out.
So then if you rig the beam to be half of the light falls on both, what's the sum of these waveforms going to be if you have a component that's at the mirror and a component at the indented? If these are different in timing by lambda over 2 when you add them, the answer is?
AUDIENCE: [INAUDIBLE]
DENNIS FREEMAN: Yeah, you get complete destructive interference. You get an out answer of 0. So the idea in the reading of them is to use interference and rig it so that 0s are represented by no light and 1s are represented by lots of light. So that's the first clever trick they do.
Then the question is, how do you focus it? When I was doing my research microscope, the research microscope-- and first off, it sits on a half ton of granite to make it all very stable. Because when you're doing the focusing, you don't want-- so the vibrations of the floor in my lab are about 100 micrometers. So it's about 100 micrometers.
And I have to have the stability of the system sub-micron. So I use a table made out of a half ton of granite. And the microscope sits on top of that. And that makes everything nice and stable.
They can't do that. So how do they control the focus without using a half-- I mean, it wouldn't work to make a Walkman that is a half ton granite, right? So what do they do to enable them to do the same kind of focusing that I was doing?
They use feedback. So the idea is to substitute feedback for precision. So rather than trying to make the parts precise, they put the imprecise parts in a feedback loop-- very powerful method. So here's what they do.
They take the laser. It's trying to focus on to the CD that has patterns in it. And so there's a beam splitter, so that part of the light goes over to the detector. Excuse me. I didn't do that right. The laser, it squirts up to here, goes straight through the beam splitter, comes back through a focusing lens, bounces off the beam splitter through another lens, and hits a detector.
So the detector is this way. And I'm showing this view then. So the detector should be there. I'm sharing a view of it over here. And it's rigged, so that these two lenses-- one of the lenses is spherical and one is cylindrical. What that means is-- you remember I've talked about microscopy in the past. Out of focus means blurred. Blurred for a spot means bigger.
As a microscope goes out of focus, the spot gets bigger. What you do with these two lenses-- because one is circularly symmetric, and the other is slenderly symmetrical, what you do is you make the beam in this direction go out of focus as it's going into focus in this direction. So because you get two lenses, one is symmetric in xy. And one has no magnification in y, but big magnification in x. You can rig it so that this dot will go out of focus horizontally while it's coming into focus vertically.
The result is that, if you move the CD closer, it becomes fat. And if you move the CD further, it becomes skinny. So all they need to do, then, is focus up and down until the dot is as close to being circular as they can get it. That's why they use a quadrant detector.
So the quadrant detector lets them calculate a plus c minus b plus d. And you would like that answer to be 0. So now you use that as your error. They use the light that hits this quadrant detector to compute this number, put that in a feedback loop that drives the stage up and down to focus.
So here's an ancient CD. The CD would be here. Here is the reading lens. Here is a platform that has an electromagnet under it that will move it up and down 100 microns. So this electromagnet is under the stage. So this whole thing goes up and down in a feedback loop that tries to make this error signal be 0, tries to make the pattern circularly symmetric. So it's extremely clever.
Then you have this pattern. So the light is twice as big. Half the photons are supposed to fit in the pit. And half of the photons are supposed to hit in the land that surrounds the pit, so that as the CD is spinning, if the spot illuminates a place that's entirely in the pit, the dot is small because of interference. And if it hits a place that doesn't have a pit, the dot is large, because there's no interference.
But then you have the problem of, how do you keep the dot reading the center of the groove? So in this technology, we kept the dot in the center of the groove by using gravity. We obviously don't want to touch that surface. So that's not the technology we use. We don't use a screw.
We use feedback. So here what we do, instead of projecting a single dot, we use a beam splitter that turns the one dot into three dots. And the three dots are positioned so that they hit two more photo sensors, one on each side of the main photo center.
And the idea is that, if the light is perfectly centered on a track, these will have equal brightness. But now if the beam is off center, one of the dots will be bright, because it hit all land, compared to the other one, which some of the light is hitting the pit. So this is just like the head tracking thing in 601. So whichever of the two photo sensors gets more light, you move the track to compensate for that. So it all works in a feedback loop.
So the idea here is the same sort of deal. You have two sensors here. And you use the error, which is the difference between the two, to move the head to the track. And the head motion is this thing over here. So here's a rack and pinion connected up to a motor. And so you have that motor servo-ed back to some photo sensors over here that keep the beam centered.
So the idea, then, is that what you get for $10 to read this thing is completely remarkable. The idea is that it's got very complicated servo mechanisms that can read very small things that even a $70,000 microscope has trouble reading. And it's stable. And you can run them, or you can jostle them around, and jog with them.
And that's all because they use feedback, rather than trying to make precision machining. And then they make the signals robust by using the kind of DT signal processing that we talked about. So anyway, the point of today was just to give it some motivation of the way 6.003 factors into technologies that you might be interested in.
And this is just one example. I could have chosen any number of different devices and they would have been similar. So I hope you enjoyed the course. I hope that you have fun with the final. Please come on Tuesday, and tell us what we should do differently next time. Thanks.