Flash and JavaScript are required for this feature.
Download the video from iTunes U or the Internet Archive.
Topics covered: Molecular Evolution
Instructors: Prof. Robert A. Weinberg
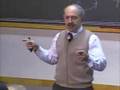
Lecture 32: Molecular Evolu...
So we're shifting gears today. We're going to talk about molecular evolution, i.e. how do we understand how species evolve, how do we understand a lot about ourselves, how human evolution is taking place over the last couple hundred thousand years. And traditionally, evolution has been the purview of people who study the morphology of organisms, and when I talk about morphology, obviously I'm talking about shape and form. And by comparing organisms, starting already 250 years ago, one began to develop hierarchies of how different organisms on the planet are related to one another.
You've seen this, undoubtedly, in high school biology.
This is the study of phylogeny, and phylogeny has traditionally been figured out by comparing the phenotypes, the morphologies of adults, sometimes embryonic development, and on that basis, attempting to extrapolate back in evolutionary time, about the relatedness of different organisms, one to the other.
And in so doing, one has been able to create, for example, family trees, here's something that Charles Darwin was already interested in, the various kinds of finches on the Galapagos Islands off Peru, in the Pacific. And here, one is beginning to organize different bird species on the basis of whether they're more closely or less closely related to one another, and to draw pedigrees, which, one imagines, describe how they evolved, one from the other. i.e., organisms which are very similar to one another must be more closely related evolutionarily, and conversely, those that appear very differently from one another, morphologically, must be far more distantly related to one another.
In fact, these kinds of morphologic extrapolations can be very misleading. So here, for example, are two kinds of eyes.
The top eye is a Drysophila eye which, to state the obvious, looks a lot different from our eyes, which is that the chordate eyes shown the bottom.
Totally different. Our rods and cones face backwards, the Drysophila Arthropod eyes, the light sensors face forward.
Everything is different. And on the basis of that, you would say that these two organisms are independent evolutionary inventions, that they've been invented on two occasions, and that they have no relatedness, one to the other, at all. But I will tell you an extraordinary experiment. You can take, there's a master gene that controls eye development in the fly, Drosophila. It's called eyeless, if you knock it out then the eye doesn't develop at all.
And you can take out of the mouse, what is apparently a related gene called small eye, so the fly gene is called eyeless and the mouse gene is called small eye. And you can put the small-eyed gene into the Drosophila genome, in a fly that lacks the eyeless gene.
So here we're talking about two different genes. The fly gene is called eyeless, the mammalian gene, at least in mouse, is called small eye.
If you knock out this gene in the fly genome, and replace it with this gene, you get a perfectly normal Drosophila eye.
It's extraordinary. Or you can do the following, you can arrange it so that the mouse small eye gene is expressed ectopically. When I say ectopically, I mean that it's expressed in the wrong place, at the wrong time.
So you can do the following experiment. In a fly genome, you can arrange it so that the mouse small eye gene becomes expressed on one of the extremities of the fly, on one of the legs of the fly. And now, on one of the extremities of the fly, an ectopic eye will develop, looks just like a Drosophila eye, but it's development is programmed by the mouse small eye gene. What I'm telling you is that these two genes are totally interchangeable, that they are effectively indistinguishable from one another, functionally they have some sequence relatedness, but in terms of the way they program development, they are effectively equivalent.
And what this means is that the progenitor of these two genes must've already existed at the time that the flies and we diverged, which six or seven-hundred million years ago, and in the intervening six or seven-hundred million years, these genes have been totally unchanged. i.e., once the gene was developed, evolution could not tinker with it, and begin to change it in different ways, ostensibly because such tinkering would render these genes dysfunctional, and thereby would inactivate them, thereby depriving the organism of a critical sensory organ.
So here we have an example, a dramatic example, of how morphology misleads us. Here we have an example of where we would say these two eyes, the two eyes I've shown you here, are so different from one another that they must, by necessity, be independent evolutionary inventions.
But in fact, genetics tells us, and these gene-swapping experiments, tell us that the two eyes descend from a common ancestral eye, a prototypical eye, whose precise morphology we can't discern anymore.
And so we begin to realize that if we really want to understand evolution and we really want to understand phylogeny, phylogeny being how the species are related to one another, we have to the DNA, and we have to begin to look not at phenotype, but we have to look instead, at genotype.
The Darwinian model is pretty much like this, the survival of the fittest. And when I say that, we imagine that we have here, a genetically heterogeneous group of organisms within a species, and this, this number of individuals in the species could be 100, it could be 1,000,000. This particular individual, by chance, acquires a mutation, or an advantageous allele, through some genetic alteration. This genotype renders this organism more fit, phenotypically, has a selective advantage and consequently, over extended periods of time, which may be thousands or even millions of years, the descendents of the organism bearing this allele now have advantage, have greater reproductive advantage, survival advantage, compared with the other individuals in the same species, and therefore, the representation of this mutant allele in the gene pool of the species becomes expanded.
When I say gene pool, I'm talking bout the common shared set of genes within the species, such as within the human species.
And so eventually, the descendents of this organism, or the descendants of an organism bearing this allele, now become overrepresented in the population, because they're more fit.
And then there can be another succession, i.
., there could be other mutations occurring subsequently.
Once again, favoring the selective outgrowth of an individual bearing this allele, or this allele. And in addition, there can be the process of what one calls, speciation.
That is to say, that if some parts of the species live in one place, and other parts of the species live in another place, geographically, they may no longer interbreed, and as a consequence, and because of the fact they're under different selective pressures, they may begin to diverge from another if, evolutionary speaking, because they're no longer actively exchanging genes within one another.
And, as a consequence, one can have new species arriving.
And what one believes, this happens over slow, slowly over evolutionary time, but it does arise, and to the extent it does, one eventually ends up with organisms here and here, which can no longer effectively interbreed with one another.
That is to say, they become genetically so different from one another, that any hybrids formed between them are, in fact, sterile, for one reason or another, if they're at all interested in breeding with one another to begin with.
And what this means is that we can begin to trace how closely or distantly related species are to one another, simply by asking how closely or similarly related are their DNA sequences?
If, distantly related organisms have very distantly related sequences, and closely related organisms must have sequences which are very similar to one another. And over evolutionary time, there's a so-called mutational clock, where one, where each species accumulates a certain number of point mutations, base substitutions in it's DNA, per million years, and the longer the two species are separated from one another, the greater will be the difference in their sequence diversity.
And on that simple basis, one can begin to construct evolutionary trees of, for example, the entire cellular life on the planet. And here's such an evolutionary tree, where what's being compared is the ribosomal RNA sequences, i.e., the small ribosomal RNA. Remember, ribosomes have two subunits, small and large, in the case of prokaryotes, it's 16S RNA, that is, it's sedimentation rate.
In the case of mammals, it's 18S. In both cases, these are small ribosomal RNA subunits. The ribosome was only invented on one occasion during the evolution of life on the planet, so one can begin to compare since all cellular life forms have life forms, one can ask how similar, or dissimilar, are the various sequences and coding, in small ribosomal RNA subunits?
And on the basis of that, one has concluded that there are actually three branches of cellular life on the planet.
The bacteria indicated here, this is not such a great Xerox, where you see a whole series of different kinds of bacteria, indicated on this tree. Sorry about the poor reproduction.
Here there's a dashed line indicating that we're talking, there's a second kingdom in the middle here, indicated by what are called archae, and the archae are also, from our point of view, prokaryotes, but they're not bacteria. They are a single-cell life form, they're often found in unusual situations, for instance, in thermal vents in the bottom of the ocean floor, some of them are able to stand high salt, some of them are able to stand high temperature, like therma fillus, therma proteus, and so fourth.
And these, their ribosomal RNAs, are so different from those of bacteria, that they've been placed in their own separate kingdom.
And finally, here are the eukaryotes, all over here.
These are all eukaryotic cells, starting here. And we, our cells, seem to be slightly more closely related to those of the Archaea, if you follow this ribosomal sequences, than they are to the actual bacteria. So, there's actually two major prokaryotic life forms on the planet. The first living organism, well if you begin to try to look back in geological record, it looks like the first living cellular life forms existed already 3-3.5 billion years ago, not so long after the planet was formed, which was between 4. and 4.5 billion years ago. And here's the whole eukaryotic tree, and if we look at the eukaryotic trees, remembering here that we're starting at 3.5 billion years ago, And we're using this evolutionary clock to determine relatedness, then we see a whole series of single-cell eukaryotes, here are their names are, these are protozoan, eukaryotic protozoan. Here's Amoeba, here are slime molds, here are cilliates, we're still at single-cell organisms.
Finally we get to multi-cellular organisms, plants, fungi, and animals, and so, all animals on the planet are a relatively recent invention. All animals, all of the metazoan, are just on this very small branch, and we know that this very small branch started around 600-650 million years ago, maybe 700 million years ago. And that was the time, roughly speaking, when we and flies last had our common ancestor, otherwise, to state the obvious, we and flies are very different.
The fact that the gene for encoding the eye has been conserved, so faithfully, over enormous evolutionary period of time indicates something else, And that is, certain genes can evolve progressively over a long period of time, because they don't encode vital functions, or they may even be sequences between genes that don't encode phenotype at all.
Imagine, for example, we have a situation were here we have a gene which encodes a vital function, like the eye, here's another gene that encodes another function, oh I don't know, a leg.
And here we have intergenic sequences. After all, as you have learned by now, more than 96% of the DNA in our genome, doesn't encode proteins, and probably isn't even responsible for regulating genes. So these sequences, right in here, can mutate freely during the course of evolution, without having a deleterious effect on the phenotype of the organism.
There's no evolutionary pressure to constrain the evolution of these genes, but if this gene over here encodes an eyes, And if this gene has been optimized in it's sequence, early in the course of evolution, that any subsequently occurring mutations will compromise it's function, and therefore there's enormous selective pressure to eliminate any organism which has begun to tinker with the sequence of this gene, by changing it's sequence.
Here, in stark contrast, there's no such selective pressure.
The organism, that is, can tinker at will with this. I don't mean literally that the organism is able to tinker with it's own DNA sequences, but the hand of evolution can change these sequences in here, at will, without having any effect on the viability of the organism on it's selective, or Darwinian, fitness, and therefore, such mutations in these, in these sequences, are neutral mutation, they have on effect on phenotype, and they will not be eliminated from the gene pool.
Again here, mutations in these vital, critical genes will be eliminated from the gene pool. So that's another one of the principles in molecular evolution that we want to talk about.
And if you follow these principles, we can not only do, draw evolutionary trees like this, which have a grand scope, a scale of three and a half billion years, we can talk, for example, about how different kinds of bears are related to one another, and on the basis, once again, of their DNA sequence.
Or, if you want, we can even look at how different kinds of domesticated animals are related to one another.
This is kind of a fun undertaking. Look at this. Why is it fun? Well it's, it's kind of an amusing idea, how often were cows domesticated during the history of humanity? How often were sheep domesticated?
Pigs, water buffalos, and horses. And what you see here is that cattle were domesticated on two occasions, probably once in Western Asia, the middle east, and once in Eastern Asia. Sheep were domesticated twice, all modern sheep following these two families here.
Obviously they share a common ancestor someway back, but most sheep either fall here or here. Pigs seem to have been domesticated twice, once over here and once over here, water buffalos twice, horses are very confusing, it looks like they were domesticated on several occasions because they're all over the map, they're not two clusters of closely-related varieties, like here and here. What others, dogs, that's recently, I forget what the number is for dogs, once.
Dogs were domesticated once, probably the earliest domestication, about 100,000 years ago. They all have one common radiating tree, here we have two radiating trees, one cluster over here, one cluster over here, with sheep, pigs, and so fourth. So we can even, so you can learn an enormous amount about even the history of agriculture, by looking at these kinds of DNA pedigree.
Here's some other interesting principles. Mitochondrial DNA passes always from the mother, so when a fertilized egg is formed, Dad gives his chromosomes, but he doesn't donate for any, doesn't donate any mitochondrial DNA. I remember visiting a friend in North Carolina in 1974, and he was looking at the mitochondrial DNA of mules and, when you breed a horse and a donkey, what do you get out? You get a mule out, or, what happens if you do it the other way? What happens if the father is a horse, and the mother is a donkey?
It's a hinny, it's actually called a hinny. So there's two ways of breeding, and the question is now, and by the way, it's not so nice to have a father being the horse and the mother being the mule.
Why? Because Mom isn't used to carrying an embryo that's much larger than she's adapted to. The other way is fine, because then she can carry a small embryo, but if Dad comes from a much larger species, then the fetus that the female donkey must carry, is larger than her womb is really evolved to carry.
So, you don't often see these hinnies around because they cause great difficulty at birth. In any case, why did I get into this digression? Glad I asked that.
The question was, where did the mitochondrial DNA come?
1974, this was still a hot question. And it turned out, the mitochondrial DNA in both the mules and the hinnies came always from Mom. There was not a trace of mitochondrial DNA from the father and, as a consequence, this begins to cause us to realize where our mitochondrial DNA comes from. So his mitochondrial DNA comes from his mother, his maternal grandmother, her mother, her mother before her, and so fourth, and the same for each one of you. And what this means is that if you look at a pedigree like this, and for example, here we have a mother and a father, girls are always round, boys are square. And here you'll see the mitochondrial DNA, it's donated to all of the children, but the fact is that these boys, when they mate, when they have offspring, they will no longer pass along her mitochondrial DNA, so it will be lost. And the only way the mitochondrial DNA can be transmitted is through one of her daughters, who in turn, have daughters. Here you see the situation where almost all of her mitochondrial DNA is lost, except for this female descendent who, once again, passes it on to her sons and daughters. Only the daughters, again, can transmit mitochondrial DNA. And there is equity in life, it doesn't often happen. Jack Kennedy said life is unfair, but sometimes it's reasonably fair, but here is the y-chromosomes, the y-counterpart. Keep in mind, the y-chromosome only passes from father-to-son, to father-to-son, and exactly the same dynamics apply. And importantly, this is critical for our thinking now, neither the y-chromosome nor the mitochondrial DNA recombines with another chromosome.
And therefore, the complexities of diploid mendelian genetics are obviated. So when you're looking at, for example, the mitochondrial DNA, you can look at the pure results of accumulated mutations, You don't have to worry about crossing-over, you don't have to worry about exchange of portions of a gene between two homologous chromosomes. It doesn't happen with the y-chromosome because there's only one of them in the cell, and it doesn't happen with the mitochondrial DNA because there's no other DNA for it to equilibrate with. As a consequence, we can begin to think about what happens with mitochondrial DNA and y-chromosomal DNA, in young and old species.
So let's talk about a recently formed species, and let's say we have a young species down here, below the illustration, and now this species, which has recently come into existence for whatever reason, hangs around for the next couple million years.
And while it hangs around, there will be random mutations, which strike the genomes of individual members of that species.
And therefore, the longer the life of the species as a whole, the more genetically diverse will become the individuals in the species, and therefore, this species will grow to have more and more genetic diversity, just because of the random stochastic mutations that accumulate in different peoples genomes in the course of the life of this species, over millions of years.
Again, keep in mind that the vast majority of these accumulated mutations will be mutual mutations, which will not affect phenotype, and therefore, they will not be eliminated by Darwinian selection.
And many of these neutral mutations, which have no effect on organismic fitness, but are simply evolutionary neutral, are sometimes called polymorphisms. The term polymorphism, -morph is once again morphology, derives from the fact that species tend to be polymorphic, we don't all have blond hair, we don't all have brown eyes. We, as a species, have a great variability in phenotype, we're polymorphic, and yet having black hair, and having blond hair, and having red hair, none of those is considered mutant, none of those is considered pathological. I.
., among the group of normal phenotypes, there's a whole series of different gradations, and these are considered normal gradations in phenotype, but at the genetic level, we talk about polymorphisms in the same sense.
Genetically distinct nucleotide sequences, which again, are not pathological, they don't create a disadvantageous phenotype.
And as a consequence, they are once again, not selected against.
Now look what happens over here. Here we have great genetic diversity, but what will happen is, for one reason or another, only a small subset of individuals constituting this species, will turn out to be the ancestors of the successor species.
Here's the next species that arises. And why will these just be the ancestors? Well, everybody else could get killed off through some plague, they might get killed off by somebody going out and purposefully killing them, or, it might just be that a meteor comes down and wipes all these guys out, and these are the only ones in here, from this small subset of the original species, who end up surviving, and who end up becoming the precursors, the ancestors, of the new species, and once again, undergoes a period of diversification. And what we have therefore, is a diversification and then a collapse of genetic diversity.
Here, this species, because it came from a small group, is initially rather, rather homogenous genetically, but with the passage of evolutionary time, once again, there's evolutionary diversification. So, an older species actually ends up being much more genetically diverse than does the younger species. If you look at two chimpanzees living on opposite sides of the same hill in West Africa, they are genetically far more distantly related to one another, than any one of us, by a factor of 10 to 15. Two chimpanzees, they look exactly the same, they have the same peculiar habits, but they're genetically far more distantly-related than we are to one-another, than I am to any one of you, or than any one of you is to one another. And what does that mean?
It means that, roughly speaking, the species of chimpanzees is, at least, 10 or 15 times older than our species are. We're a young species, chimpanzees probably first speciated three or four million years ago, if the paleontological record is, is accurate. Paleontology is the study of old, dusty bones, so you can begin to imagine when chimpanzee bones become recognizable in the earth.
So a paleontologist says that, that chimps aren't that old, and it begins to suggest that our species is only about 200, 00 years old, at the oldest. Now you say 200,000 years is a long time, but it isn't so long because I started out this discussion talking about 3.5 billion years, 3.5 times ten to the ninth, and now I'm talking about two times ten to the fourth.
Is that right? No, two times ten to the fifth.
Four orders of magnitude difference. So that means that our species, we went through a bottleneck about 200-250,000 years ago, and because that is so recent, we haven't had a chance to actually acquire much genetic diversity. We're actually very closely related to one another, although to talk to people, you'd think we were all very distantly related to one another.
Here's another interesting notion, which also figures in, and that is, what happens in the genetics of small populations?
So here we started out with eight individuals, and let's assume for a moment, that this population has a steady size, i.
. it doesn't increase or decrease over the course of several generations. And what that means is that each couple will, on average, leave behind two children, and those two children will breed, and each of them will, couples in the successor population, will leave behind two children. And what you see already, in such small populations, is that for example, this male here has two girls, and right away, to the extent he had an interesting y-chromosome, that y-chromosome was lost from the gene pool. This girl, here, had an interesting mitochondrial DNA, but right away that's lost, because she has, she has just two boys. And what you see, in very rapid order, in small populations, there's a homogenization of the genetic compliment, just because the alleles are lost within what's called, genetic drift.
And as a consequence, very rapidly, there becomes homozygosity at many loci in very small populations.
A real-life situation comes from Mutiny on the Bounty, where Fletcher Christian ends up getting shipwrecked on, what island was it, Pitcairn Island, which is somewhere on the South Pacific, South Atlantic, I forget where. Anyhow, today if you go to Pitcairn Island, almost everybody is called, almost everybody has a family name, Christian.
Why? Was it that he was more studly and fecund than everybody else?
Probably not. What probably happened was, in the same dynamics that dictates the homogenization of y-chromosomes, dictates the homogenization of family names. So, if you isolate people in a small demographic isolate, like an island in the middle of the ocean, over a period of generations, roughly equal to, I think, twice the number of individuals in the steady, state population, everybody will have the same family name, because the other family names will, by chance, in a small population, just be lost. On my father's side of the family, I have hundreds of cousins with my family name, and on my mother's side of the family, not a single one, just as an example of this kind of trait. Now keep in mind that this evolutionary diversification can also affect the y-chromosome, so therefore, there are different y-chromosomes across the face of the planet, which can be distinguished, not because they're better or lesser y-chromosomes, in terms of the phenotype of maleness, but because they've accumulated polymorphisms over a period of time. They may be single-nucleotide polymorphisms, but these single-nucleotide polymorphisms can be used to determine how closely, or distantly related, are individuals to one another.
Let's look at the mitochondrial DNA of women in western Europe, and if you look at the mitochondrial DNA of women in western Europe, you find that they only have, how many different things there?
One, two, three, four, five, six, seven, there's seven basic types of mitochondrial DNA that are found in western and northern European women. And what that means is, inescapably, people who live in modern-day Europe, descend from seven women who had these respective mitochondrial DNA sequences. When did those seven ancestors live, well we don't really know, probably between 10-15,000 years ago.
But, the western-European population descends from an stunningly small number of founders.
Now clearly, DNA sequencing is terrific, but it's not good enough to know the names of those women, so I can tell you that they were not named Velda and Jasmine. [LAUGHTER] Anyhow, but here you can see, here you can, now obviously, these women in turn were related to one another, you can ask, you can do another kind of question. How much are all of our mitochondrial DNA are related to one another, how distantly related are they to one another, given the rate of evolution of mitochondrial DNA sequences?
And if you ask that question, the answer is that we all had a common ancestress who lived about 150,000 years ago.
All of us trace our mitochondrial DNA to her. Does that mean that there was only one woman alive there, she's called, Mitochondrial-Eve, again, we don't know her name. Does that mean there was only one woman alive, well it doesn't mean that at all because of what I just told you, in small populations the proto-human population.
I just told you that certain polymorphisms die out because of this genetic drift, because of these stochastic events.
And so, the founding human population could have had 20, 50,100 individuals in it, but one woman's mitochondrial DNA happened because of these accidents to dominate, so that now, all of us have the same, are the descendents of her mitochondrial DNA. Clearly, in the intervening time since 150,000 years ago, accumulated mutations have, have altered subtly the mitochondrial DNA genome, so there's polymorphisms, And so one can make, one can drive phylogenies of different kinds of mitochondrial DNA, and look at the relatedness between different clades, different groups, of women in modern-day Europe.
70% of Finish men, in Finland, have a y-chromosome polymorphism that is otherwise virtually unheard of in the rest of Europe. 70% of Finish men, now what does that mean?
Well, to me to means that those 70% of Finish men descended from a common ancestor, a male who lived around, if you look at the sequences, who lived around two or three thousand years ago, and who, for some reason, became the ancestor of all the people living in modern Finland.
That's extraordinary. There's four million people living in Finland today, and the males all have their inherit, inherit their y-chromosome from that man, we don't know exactly where he lived, But obviously the modern Finish population descends from a very small founder-group who came into what we call, modern Finland, relatively recently, maybe two-two and a half thousand years ago, and thereafter, did not freely interbreed with the rest of the European population.
How do we know that? Because that y-chromosomal polymorphism is not present elsewhere, it's only present in Finland. So it was a genetic, and obviously linguistic, isolate.
So where do we all come from, all of us human beings? How closely related are we to one another?
Here's, here's a measurement of the distances between different mitochondrial DNA's from different branches of humanity.
And what you see is something really quite extraordinary and stunning. Here, you'll see that the people, the non-African lineages here and here, are actually relatively closely related to one another. But if you look at the people who live in Africa, down here, there is enormous genetic diversity. Look how far these evolutionary branches reach back, look how long these are. The distance of these branches, of these roots, determines how far, how distantly related these individuals are, one to the other.
And on the basis of that, and on the basis of a lot of other auxiliary genetic information, we can conclude that Africa was the site where genetic diversification was generated during human evolution. And that what happened, as a consequence of that diversification, is starting over the last 40, 50, 60,000 years ago, different populations, different sub-populations, small, isolated sub-populations, migrated out of Africa, took a very small sub-set of the polymorphisms with them, and became the founder-populations of a whole variety of whole different modern-day populations. These populations here are largely Mongoloid, these populations here are largely Caucasian, and here, we see that in Africa there's enormous genetic diversity. And by the way, all the genes that are present here, the alleles that are present here, can also be found in Africa, but in relatively small proportions in Africa. And we know this kind of diversity exists both for the mitochondrial DNA, and here's for the y-chromosomal DNA, again, we look for polymorphisms. And this is not a very good overhead, again, the reproduction was not very good, but what I'm showing you is that the evolutionary, the depth of these evolutionary branches is enormous in Africa, yet in other parts of the globe, people are much more closely-related to one another.
Some people argue on the basis of the genetic-relatedness of western and northern Europeans, that the modern European population is largely descended from about 20 couples that moved into Europe about 10,000 years ago, eight to ten thousand years ago, at the time when agriculture was introduced into Europe from the middle east, just on the basis of looking at these y-chromosomal sequences. And so, we human beings arose, even though we are reasonably distantly related to one another on this graph, keep in mind that we as a species, are enormously close to one another because of the youth of this, of our species.
If you look at our, the time of this diversification was probably sometime between 80-100, 00 years ago, so how did it all happen? We can even figure out the history of humanity by beginning to look at these different kinds of polymorphisms.
A long time ago, individuals went out from Africa, maybe starting 100,000 years ago, maybe starting more recently, and went across the southern rim of Eurasia, and we know already, we find archeological remains of Aborigines in Australia between 40-60,000 years ago. And by the way, those people are very distantly related to the rest of us, having left and not intermingled with the rest of humanity for a very long period of time. There were Aborigines in Australia, already at a time when our ancestors, to the extent we had ancestors in Europe, were still battling the Neanderthals, who only died out 30, 00 years ago. You may know by the way, you may have read in the newspaper, about a month ago, they discovered skeletons of very small people on an island Indonesia. In fact, those were probably not even homosapiens, those were probably a precursor species, because we know over the last two million years, there have been hominoids, look like human beings but are precursors, who might migrated out of Africa, who dispersed throughout Asia, and who eventually became extinct, So that the only modern human who exist are the descendents of this out migration that began about 100, 00 years ago. We know that about 15, 00 years ago some of these people ended up going over here, to crossing in four different waves of migration, you can see it from the DNA, into the western hemisphere.
Amerindians, that is, American Indians, Native Americans, are genetically rather homogenous. Why? Because they all descend from very small founder populations that came into the western hemisphere relatively recently. And there's enormous genetic homogeneity among different subgroups of individuals here in South America. Speaking of South America, if you look in some parts of Venezuela, what you find is that the mitochondrial DNA is largely of Indian-origin, but the y-chromosomal DNA is largely of European origin.
So, what happens there, that's a testimonial to the tragic fate of the Indians, where the conquistadors from Spain came in, killed all the men, and took all the women, to be their brides. How else can you explain the fact that there's no Indian y-chromosomes, there's all, there is instead only European y-chromosomes. And here you can begin to see what happened here in New York, as well. 40,000 years ago people started trickling into Europe, and they hung around there for the next 30,000 years, pretty much on their own.
The remnants of those people who came in, we know from DNA, are the Basques who live in northern Spain, who speak, by the way, a non-indo European language.
They're the relics of this initial settlement by modern humans of Europe, starting 40, 00 years ago. And they had the continent for themselves for the next 30,000 years, until this new founder population came in, about 10, 00 years ago. Here's the names of the girls who were in that group, Ursula and Katrine and Zenya, Tara, Jasmine, and Velda, and they became the modern agriculturalists, and swamped out the people who were there 40,000 years ago, who now only survive as a relic population. Here's a fun story I like to tell each year, and it's about the Cohen and y-chromosome, and you'll see what an amusing story this is, just from genetics.
Now the name Cohen, in Hebrew means, a high priest, and you've heard people named Cohen, it's not such an uncommon name among the Jews. And it says, in the Bible, in Genesis and Exodus, that all the high priests in the Bible are the descendents of Aaron, the brother of Moses. And it's also been the practice for the last 3,000 years, that the only person who can become, the only male who can become a Cohen, is the son of a Cohen.
In other words, you cannot be adopted into a family and acquire the name Cohen. And if that's all true, and if the Bible is true, and Aaron lived 3,000 years ago, whatever his name was, then it should be the case that all male Cohen's should have the same y-chromosome, right?
Because they all descend, their family name is Cohen, they could only get it from their father, they could only get their y-chromosome from their father, so they should all have the same y-chromosome. Of course, you say that can't really be the case, because we know in this country, in this country, between five and ten percent of people, on average, are sending Father's Day cards to the wrong person.
What does that mean? Non-paternity.
When you do genetic counseling of family these days, one of the strictures is, that you never tell the family if the children have genetic polymorphisms that don't match that of the person whom they think is their father. They don't look like their, the person whom they regard as father, but that's always assumed to be a role of the genetic dice. So, how is that relevant? Well, let's talk about this descent from Aaron, who lived 3,000 years ago.
We're talking about the y-chromosome being passed from one generation to the next, just like the family name. So what happened, what would happen if sometime over the last 3, 00 years, Mrs. Cohen had a dalliance, had an affair, with a television repairman, or the milkman, or the mailman, and never told Mr. Cohen? The y-chromosome, which her son thought he was getting from Dad, wouldn't be coming from Dad, it'd be coming from this other, the milkman or the mailman, and it wouldn't be a Cohen-y chromosome unless, by chance, the mailman or the milkman also happened to be a Cohen, [LAUGHTER], it could happen.
But the chances are, roughly speaking, Cohen's are only four percent of all Jews, so the chances are against that happening. OK, so they did this experiment, and this is really astounding experiment. They went, the story is they went to a beach in Tel Aviv, I don't know whether they actually did that or not, and they picked up, they picked up 100 male Cohen's who were Ashkenazi , Ashkenazi means their ancestors came from central Europe, over here. And they picked up 100 Sefardi Cohen's, and the Sefardi Cohen's come from Spain, North Africa, Egypt, Yemen, Iraq, Iran, Uzbekistan, Central Asia. And the last time that the Iraqi Cohen's and the Ashkenazi Cohen's were interbreeding, were about 500 BC, at the time of the Babylonian XL, so they've been apart a long time.
And they looked at their y-chromosomes, and what they found was that 70% of the y-chromosomes of these male Cohen's, 70% of the Cohen's shared the same y-chromosome.
Well, the same y-chromosome was present only in 15% of non-Cohen, Israeli Jews. Now think about that for a second. 70% of these men had the same y-chromosome, of course they didn't know they had the same y-chromosome, all they knew was that they had the same family name. And what that means, inescapably, is that over a period of two or three thousand years, it was hard to trace with exactitude when the common male ancestor lived, over a period of two or three thousand years, somehow the milkman and the mailman stayed away from Mrs.
Cohen, or Mrs. Cohen was unusually virtuous.
Because keep in mind, any single affair with the milkman or the mailman, over 3,000 years, would've broke this chain of inheritance, any single incidence of non-paternity. It's a really astounding story, and it's hard, there can be no artifact to it, there's no bias in it, there's no other way to explain it.
And you can begin to find similar stories of families in England, where males are tenth cousins of one another, they have the same family name, and they also have the same y-chromosome.
The most amusing commentary on this stems from a tribe that lives in southern Africa, and these people are called, Lemba, L-e-m-b-a. And the, the myth of the Lemba is that they descend from Jews who came down from the north, Jewish traitors.
So just for the hell of it, some geneticists went down and drew blood from the male Lembas, and there's four casts of Lembas, there's the ruling class, there's the warriors, there's the farmers, the merchants, I don't know. And what they found was that all members of the, almost all members of the ruling cast among the Lembas, had the same y-chromosome, and the y-chromosome had exactly the same polymorphisms of the Cohen y-chromosome. No one in the other, no males in the other three casts had, or very few, had otherwise this y-chromosomal polymorphism. Go figure, I don't know what's going on.
Now did those people look Jewish? Well, they looked like everybody else around them, because if there was a Mr.
Cohen who came down there, three or four hundred years ago, and married in, he obviously married one of the local population.
And there were no other people around from, coming in from the north, to marry to, so that 99% of the males in this ruling cast, who have the Cohen y-chromosome, 99% of their genes come from the local population, the only thing they inherited from Mr. Cohen was the y-chromosome. Where else would they get their genes? There wasn't a massive migration from the middle east down to the Lemba tribes, probably just one man came down selling trinkets, or who-knows-what, television sets, or VCRs, sometime over the last three or four hundred years. And somehow, for reasons that we have no idea, he became the ancestor of this cast of people in this tribe in the middle of Africa. And so you have stories that you begin to pick up, which are stranger than fiction, some of the weirdest stories that you've ever heard of in your life.
Imagine having 3,000 years of uninterrupted transmission from, without a single case of non-paternity.
It didn't happen all the cases, because I didn't say 100% of the Cohen men had it, on 30% of the occasions, there must have been some snipping of this chain of transmission.
And keep in mind that this chain of transmission happened over a period of enormous political and upheaval, over the last 3,000 years. The middle east, and Europe, and North Africa, have not been tranquil places over that period of time. Enormous population dispersal, and confusion, and displacement, and yet we now begin to look, by looking at the DNA, we can begin to see all kinds of really interesting things.
Next, on Friday, Eric is going to talk with you, I believe, on Monday, as well. And Wednesday, we're going to talk about a related topic, which is, how do all, how do these human genetic differences have implications for the way we think about one another, and the way that we will develop?
See you then, a week from today.
Free Downloads
Free Streaming
Video
- iTunes U (MP4 - 139MB)
- Internet Archive (MP4 - 139MB)
Audio
- iTunes U (MP3 - 12MB)
Caption
- English-US (SRT)