Flash and JavaScript are required for this feature.
Download the video from iTunes U or the Internet Archive.
Topics covered: Virology/Tumor Viruses
Instructors: Prof. Robert A. Weinberg
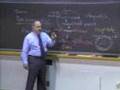
Lecture 21: Virology/Tumor ...
Good morning class, nice to see you again.
I trust you had a relaxing weekend and a Happy Halloween, whatever a Happy Halloween is. You recall that last time we were talking about the process of cell transformation, and recall, what I said was that transformation represents the conversion of a normal cell into a cancer cell.
In fact, there are a variety of traits of a cell which suggest it's a cancer cell, it changes its shape, it can get along with [foralized? growth factors. Normal cells require tethering to the bottom of a Petri dish in order to grow.
Cancer cells, you can put them into a semi-solid medium, like agar, and cancer cells will often grow like this, as colonies in suspension, without direct tethering, without direct adhesion to a solid, underlying substrate.
And that, that's a trait of cancer cells, the phenotype of cancer cells, is sometimes called, anchorage independence.
But ultimately, the best litmus test, whether a cell is truly transformed, is tumoraganisity, i.e. the ability of a cell, when plucked out of a Petri-dish like this, and implanted into a host mouse, to actually grow into a tumor.
So there are various gradations of becoming transformed, but tumoraganisity is the ultimate arbiter of whether or not a cell is truly transformed. Now, you'll recall from our conversation last time that if you put a transformed cell, a mixed a mono-layer of normal cells, that the transformed cell will overgrow the mono-layer, it will have lost contact inhibition, and that when viewed from above, such a Petri-dish yields a thick clump of cells, which is called a focus, plural, foci. And I will tell you that beginning in the late 1960's, one began to use a variety of different techniques, with which to transform cancer cells into normal cells. One of the techniques one used was to apply chemical carcinogens to cells, and keep in mind that we're reserving the word, carcinogen, for a chemical or a physical agent that causes cancer. Ultraviolet radiation is a carcinogen, as are x-rays, and there are many chemical carcinogens, such as those in tobacco smoke.
And certain experiments in the early 1970's began to reveal that one could get foci of transformed cells, by applying chemical carcinogen to the cell in-vitro, and when I say in-vitro, I mean growing here, in the Petri dish. And, in fact, one could begin to use a whole variety of different types of carcinogens, chemical carcinogens, and what seemed to be shared in common between all these carcinogens was that they were all mutagenic.
By mutagenic, I clearly mean the ability to inflict damage on the genome of the cells that were being exposed to these various compounds.
In fact, one could draw an interesting correlation because many of these mutagenic compounds had, also by chance, been tested in laboratory animals for their carcinogenicity.
And so plots were derived in the mid-1970's, between the mutagenic potential of a compound, and the carcinogenic potential of a compound. And when I say a carcinogen, how carcinogenic is a compound, I mean, how many milligrams of this compound does it take to make a tumor? And so, what one could do, is plot over a log-log scale, how many milligrams of a given compound was required, or micrograms, to make a tumor in a rat or a mouse.
And, at the same time, how mutagenic were these compounds, i.e. how potent were they in their ability to inflict damage on the genome? Because it turns out, that if this log-log scale is by orders of magnitude, powers of ten, compounds range over at least five or six orders of magnitude in their potency in inflicting mutations, and similarly, in their potency in inducing tumors in mice and rats.
And what one found was the following, that there was a log-log relationship that was roughly linear, but there was violations to this.
Some compounds were extraordinarily mutagenic, they could create mutations in very low doses, and at the same time could create tumors, they were carcinogenic in very low doses.
Other compounds required an enormous amount of material in order to induce mutations on the abscissa, and an enormous amount of material in order to induce tumors on the ordinate. And this log-log relationship over five orders of magnitude, suggested the following obvious idea, that carcinogens are mutagenic, and to the extent that carcinogens are able to induce cancer, they do so through their ability to inflict mutational damage on the cells within certain target tissues. And this, therefore, obviously suggested the notion that within cancer cells, as we said last time, there are mutant genes, and that these mutant genes are moreover instrumental in conferring the transformed phenotype on the cells that carry these mutant genes.
In the, in the late 1960's and early 1970's, a man named Howard Temin, began to use a virus called Rous sarcoma virus, which had first been discovered in 1909 by a man named Peyton Rous.
Rous was then a professor at the Rockefeller Institute, later the Rockefeller University, in New York. A Long Island chicken farmer brought in a prized hen of his who had been growing a big muscle tumor, or sarcoma, in her breast muscle, and asked Rous, the famous chicken doctor, if he could cure this chicken, and Rous said, thank you, cut off the hen's head, extracted the tumor, and ground up the tumor, and after having homogenized the tumor, passed the homogenate through a filter. And this filter would trap all cellular material, but it would allow non-cellular material, or material that was smaller than the size of a cell, to pass right through. And so, therefore, Rous took the material that passed through the filter, which you can call the filtrate, and he injected the filtrate, which passed through the filter, into a young chick. And what he observed thereafter was that chick soon came down with sarcoma after a period of some months, and when he ground up the tumor in that chick, and once again injected into another chick, he once again got a tumor.
The fact that the agent, which was inducing the cancer, and could be transmitted from one animal to another, from one chicken to the other, was filterable, suggested it was extremely small, and at the time one had already begun to appreciate the fact that there were sub-cellular infectious agents, which we now call viruses. And Peyton Rous made this very important discovery in 1909, 1910, and in 1965 he was awarded a Nobel Prize for this. It's a rather long wait, wouldn't you say? So he only had to wait 55 years, anyhow, he died a happy man, we can only presume. Now, what's interesting about this is the life cycle of Rous Sarcoma virus.
As we will discuss in greater detail later, viruses are sub-cellular particles, they don't have their own energy metabolism, and they parasitize on the macromolecular machinery of the cell that they infect. And therefore, what we can imagine here is the following scenario, which actually happens to be true.
A virus particle, which is vastly smaller than a cell, enters the cell, the virus particle carries into the cell its own genome, and this genome, in this case of Rous Sarcoma virus, is single-stranded RNA, and this genome, which is carried into the cell, carries the information for making more virus particles.
And what happens is, in the case of Rous Sarcoma virus, as Temin later speculated in a speculation that caused him ridicule and ostracism for many years, that the single-stranded RNA of Rous Sarcoma virus, once it gets into the cell, is reverse-transcribe, i.e. copied into DNA molecules.
So now we have double-stranded DNA, a double-stranded DNA copy of the viral genome, and this double-stranded DNA molecule, which came to be called a pro-virus, then became integrated into the host chromosomal DNA. So here's the host-chromosome.
And now, the pro-virus, which I'll depict here in the middle, in white, became physically inserted as a double-stranded DNA molecule, it was slipped right into the genome. We now realize that any, any of tens, of hundreds, of millions of different sites in the genome of the host cell. And this pro-virus, once established or integrated into the genome, could thereafter, function essentially like a cellular gene, i.e. from a molecular-biological perspective, it was indistinguishable from a cellular gene, it was double-stranded DNA, it had a promoter it carried in a promoter with it, and it had a polyadenylation signal, and therefore, this pro-virus thereafter, could use, or parasitize, the host-cell RNA polymerase 2, to make viral messenger RNA, on the one hand, and progeny-genomic RNA.
Now when I say genomic, I'm not talking about the host-cell genome, I'm talking about the viral genome. How big is the virus?
Well, it's about nine or ten kilo-bases in length, so it's genome, obviously, is vastly smaller than the 3.
mega- bases that constitute the haploid human genome.
The viral mRNA, once transcribed in the nucleus, and exported to the cytoplasm, could make viral proteins, and the viral proteins could then be used to encapsidate, and when I use the word encapsidate, keep in mind, I never use a simple word when a polysyllabic one is possible. So when I, when the viral proteins encapsidate the viral-RNA, you get a virus particle like this, which has virus proteins on the outside, almost on the outside, viral RNA in the middle, single-stranded RNA, and this single-stranded RNA comes from the transcription of the pro-virus that has now been integrated into the genome.
Integration is an important concept here, i.e. it becomes covalently linked. And this suggests to us that the virus actually encodes several specialized proteins.
One set of specialized proteins is required for the reverse-transcription, and in fact, the virus actually carries with it, into the cell, not only its RNA, but also, reverse transcriptase. So if you isolate the virus particle, it has, in addition to this coat, it has within it already reversed transcriptase, molecules, so that the moment that this virion, a virion is a virus-particle.
The moment that this virion, or virus-particle, penetrates into the cytoplasm of the cell, there is then, immediately, an abundant supply of the deoxyribonucleoside triphosphates, the process of reverse-transcription can begin, the double-stranded DNA can be produced, exported to the cytoplasm, where a second viral protein is responsible for integrating the resulting double-stranded reverse transcript into the host-cell genome. Again, that's a highly specialized function. The forward transcription, we just talked about reverse-transcription, but the forward-transcription to make progeny RNA, obviously can rely on the host cell polymerase, the virus doesn't need to make that. The viral mRNA can be translated by host cell ribosomes in the cytoplasm, the virus doesn't need to make that. Some of the viral aren't, the new viral RNA, is genomic RNA, which as I say, becomes encapsidated to make progeny virus particles. And a cell, which is infected in this way, can suffer two fates. It could be, as is in the case with many viruses like this, that the viruses, that the cell is not actually killed by this infection, i.e.
that the cell can tolerate an infectious, an infection like this, and therefore, if you look at such a cell, for days and weeks later, it will be producing virus particles, which are being released from the cell, continuously, continually released from the cell, and are able then, to pass and infect yet other cells.
The alternative to this is what is called a cytopathic effect, and the truth of the matter is, many kinds of viruses, when they go and infect a cell, and they produce their progeny, they end up killing the cell that they've infected.
So, for example, when we get infected by a cold virus, which has a different metabolism than this one, by the way, then the cells that are infected and produce progeny-virus particles are rather quickly killed, as a consequence of the infection, which is why we have damage to our nasal mucosa.
But in this case, in the case of Temin's virus, actually RSV, Rous Sarcoma Virus, that isn't the case. And therefore, in fact, one has RSV particles that are continually being released from the cell. If one looks under the electron microscope, at the surface of a virus-infected cell, one sees structures like this.
Where here is in the green is the lipid by-layer, the plasma membrane of the cell. And here, in the middle, is a viral-protein capsid, a protein capsid like this, encoded by the virus, and carried in the capsid is actually, are actually, the viral-RNA molecules, and the reverse-transcriptase molecule. And in saying that, what I mean to suggest to you, is that actually the viral particle, the virion, is slightly more complicated than I represented it to be. As the virus, as progeny particles are made, they are pushed out through the plasma membrane of the infected cell, on which occasion, they become enveloped with a layer of plasma membrane.
And this layer of plasma membrane is actually stolen as a patch from the plasma membrane of the infected cell. So in truth, actually, a virus particle like RSV, has some membrane around it, it has a protein-capsid encoded by the viral mRNA, and in the middle it has RNA and reverse transcriptase molecules.
Note, by the way, that most viruses actually, everything that the virus carries, the virus has encoded in it's own genome, in this case, the virus has stolen, has absconded, with a patch of plasma membrane from the infected cell.
Now what Temin observered, several other people before him had done so, was that when he infected a mono-layer of chicken cells with Rous Sarcoma virus, he was able to in fact, observe the appearance of foci of transformed cells.
And he observed that these foci of transformed cells actually released Rous Sarcoma Virus. So, the infection by Rous Sarcoma Virus, had led to the production of progeny virus particles, which we kind of expect of a virus. Keep in mind that if a virus can't produce progeny, it's out of business.
Or, to put it another way, the only thing a virus is really interested in, is making more copies of itself.
So, the cells in these foci of transformants were transformed, but they were also releasing progeny virus particles.
And if you took these virus particles, and he could isolate them away from any contaminating cell, the way Peyton Rous did, just by filtering them, or the filter will trap all of the cells, and allow the much tinier virus particles to pass through, then he could take these virus particles and infect another plate of cells, and once again, he would get foci of transformants.
And therefore, this virus was actually bipotential, it could do two things. It could replicate, here I've been talking about the life cycle or the replication-cycle of the virus, on the one hand, and on the other hand, it could transform cells. And subsequent work demonstrated that actually, the replicated functions of Rous Sarcoma Virus, on the one hand, and the transforming functions of Rous Sarcoma Virus, on the other hand, were encoded in separable genes.
For example, Howard Temin was able to demonstrate, and others later, that one could get mutants of RSV that had lost the ability to transform cells, but could still replicate perfectly well.
And there were yet other mutants that had lost the ability to replicate, but could transform perfectly well.
And so there were two classes of specialized genes, one involved in replication, the other in transformation. In 1975 and 1976, the laboratories of Harold Varmus and Mike Bishop, at University of California, San Francisco, or UCSF, as it's called in the trade, began to exam the origin of the viral transforming gene.
Now the viral transforming gene, because it was assumed there was only one of them, the genome is so small, it only has about ten kilo-basis, and only enough room for three, or four, or five, genes in it, not a hundred or a thousand, the viral transforming gene came to be called SRC, S-R-C. And what they observed was the following, they made a radio-labeled probe, which was specific for the SRC gene.
And they could use the radio-labeled probe to anneal to two kinds of viruses, wild-type Rous Sarcoma Virus, and a deletion mutant, I'll use the Greek -delta, a version of Rous Sarcoma Virus, which was lacking, which apparently through process of genetic deletion, was lacking the ability to transform cells. And that loss of ability to transform cells was ostensibly due to the lesion of it's genome of the SRC gene.
And what they observed is that the SRC, the radio-labeled SRC probe, as one would've hoped, was able to anneal to this wild-type genome, but it couldn't anneal to the deletion mutant of RSV, which had lost the SRC gene through a process of genetic deletion.
So, so far, so good. By the way, the fact that the SRC gene could transform cells, led to it's being called, an oncogene. The term "oncos" in Greek means, a tumor or a lump, an oncogene therefore, was a cancer-causing gene.
And therefore, Rous Sarcoma Virus possessed at least one cancer-causing gene, or oncogene. Now the mind-blowing result that happened shortly thereafter, was the following.
People in the Varmus-Bishop lab began to look for the origins of the SRC oncogene of Rous Sarcoma Virus. It turns out that the vast majority of genes that have been in virus, that are present in viral genomes, have been in viral genomes, as far as we know, for the last billion years. i.e. we have every reason to think that the evolutionary origins of viruses can be traced into the distant past. It could even be the case, some people think, some perfectly sane people think, that this whole retro-virus life cycle that I just told you about, recapitulates one of the earliest stages of cellular evolution on the planet. People believe now, with ever-increasing conviction, and keep in mind, class, people who are convinced of something are usually wrong, in a loud voice.
But people believe with ever-increasing conviction, that the first cells on earth actually had RNA genomes, rather than DNA genomes, and that the invention of double-stranded DNA genomes in cellular life forms, came later. And if it did, then the conversion from an RNA to a DNA state is reflected in the modern life cycle of Rous Sarcoma Virus, and similar viruses, which as you may know, have come to be called retroviruses, simply because they transcribe their nucleic acid backwards.
So, Varmus and Bishop were interested in the origins of the SRC oncogene that was carried by Rous Sarcoma Virus.
I say, well it probably, the Rous Sarcoma Virus, had antecedents, which existed thousands and millions of years ago, and carried the SRC oncogene. But the fact of the matter is, the SRC, the Rous Sarcoma Virus, had only been isolated once in the 20th century, when this very trusting, and caring, Long Island chicken farmer came in to Peyton Rous, hoping that Rous would cure his chicken, rather than cutting the chicken's head off. So what happened, then, was the following. They used this radiolabeled probe to look at the DNA of infected, RSV-infected chicken cells, and uninfected chicken cells. So they probed the DNA of the infected chicken cells, with this radiolabeled probe, and they probed the DNA with uninfected chicken cell, of uninfected chicken cells, once again with this radiolabeled probe. And what they, what they expected to find was the following, it's obvious, in uninfected chicken cells you don't find any SRC, and in infected chicken cells, you do find SRC, because the SRC gene has been brought into the infected cells by the infecting viral genome. Stands to reason, right? Shouldn't be any in the uninfected cells, after the cell's infected, now they have a SRC, at least one copy, they may have multiple copies of the SRC oncogene, because I haven't really dictated how many pro-viruses should be integrated into the genome of an infected cell. And what they found was puzzling, and eventually mind-blowing. Because they found that in the DNA of uninfected chicken cells, they could find a SRC gene, and these uninfected chicken cells had never experienced Rous Sarcoma Virus in any form, whatsoever. And as a consequence, they began to develop a theory, a model, which turned out, actually, to be right on, and the model was as follows, that there was a retrovirus, like Rous Sarcoma Virus, that was the precursor of RSV, and this retrovirus had replication genes, but it lacked a transforming oncogene.
This retrovirus went into a chicken cell, and when it emerged from the chicken cell, it carried not only the replication genes, but now the SRC oncogene. It had acquired a new gene, which it could then use to subsequently transform other cells that it had infected. And this, itself, turned out to be absolutely right. This SRC gene was of cellular origin, and in fact, homologs of the SRC gene, were present in all vertebrates, in all chordates, in all metazoan, there's even a distant homolog of the SRC gene that's present in sponge cells, which are obviously rather primitive.
So this SRC gene is not a recent invention, it's been sitting around in the eukaryotic genome, at least in the genome of metazoan and its precursors, for a very long time.
It was kidnapped, picked up by the Rous Sarcoma Virus, and subsequently exploited by the virus to transform cells that it happened to infect. And this acquisition and activation of a gene was obviously a rare event, because RSV, as I've just told you, was only picked up once, was only generated once.
It didn't exist in nature, and Rous Sarcoma Virus was never seen to, go from one chicken flock to the other, like most infectious agents. The ecology of Rous Sarcoma Virus is not so much of interest to us, what is of greatest interest to us in our discussion today, is the following notion that, within the normal genome of a chicken cell, there exists a normal gene which came to be called a proto-oncogene, a precursor of the, A proto-oncogene which resides in normal chicken DNA, and the fact that the proto-oncogene is highly conserved, evolutionary, dictates to us that this proto-oncogene, this SRC proto-oncogene, must mediate essential functions, otherwise it wouldn't long ago been lost. In fact, just to repeat myself, virtually identical copies of the SRC oncogene, proto-oncogene, excuse me, lie, can be found in the genomes of all vertebrates. So, a proto-oncogene is a normal cellular, growth-regulating gene, which, on this occasion, became activated, and subverted, and converted into an active transforming gene, i.e. an oncogene. So the term -proto in this case, implies a normal gene, which has the potential, under certain circumstances, to become an active oncogene. In the years that followed, it's been almost 30 years now, more than 30 proto-oncogenes have been discovered, by looking at retroviruses like RSV.
SRC is not the only proto-oncogene that lies in our genome, and therefore, we begin to appreciate on the basis of this, that our genome carries a whole repertoire of these growth-regulating genes that, when a retrovirus happens to swoop in, can be activated into active oncogenes, they can be converted into active oncogenes, and thereafter, they can induce cancer. And this obviously leads to the suggestion that the seeds of human cancer don't lie, necessarily, on the outside of cells because most kinds of human cancers are not caused by infecting viruses. That was a puzzle that was already apparent in the late-1970's. If Rous Sarcoma Virus, or similar viruses, could not be invoked to explain many kinds of viruses, how could one get cancer? And this work suggested an obvious solution.
Let's imagine that there are a repertoire of a dozen, or two-dozen, or three-dozen, proto-oncogenes that reside in our normal genome, their purpose there is not to create cancer, their purpose is to regulate normal cell proliferation.
These genes, being genes, are subject to damage, to mutation, and therefore we can imagine that in cases of human cancer, where there are no viruses involved, there can be genetic alteration of the DNA sequences of a proto-oncogene that converted into an oncogene. i.e chemical changes to the DNA, mutagenic changes to the DNA, can mimic the conversion of a proto-oncogene to an oncogene, without any virus. There are other ways by which you can skin this cat. And one experiment to demonstrate that is the following. Did we talk at the end of last time about the guy who got a bladder carcinoma after 40 years of smoking?
I'm glad we did. Good. So, let's say this person gets a, has a bladder carcinoma, and he got the bladder carcinoma, and it was called an EG bladder carcinoma, and he got it for reasons we described last time, and I can't imagine you have any illusions about whether smoking is good or bad for you, after last time. But anyhow, so, EG bladder carcinoma, pig DNA from the tumor, so we make tumor DNA.
And now, and by the way, we presume correctly that viruses have nothing to do with this particular cancer, with the development of this cancer, and by the way, the development of a disease is another wonderful polysyllabic Greek word, pathogenesis. Pathogenesis means the study of how a disease is caused, what generates the disease.
So the pathogenesis of bladder carcinoma has nothing whatsoever to do with any viruses. Maybe it had to do with the fact that cigarette smoke mutated genes, mutated, proto-oncogenes in the DNA of Mr. Jones, that happens to have been his name, or his pseudonym, who knows, Mr. Jones' bladder cells.
So one takes tumor DNA here, and one uses the procedure of transfection, where you take the DNA, make a DNA, and you put it into normal cells, by a gene transfer, or a transfection procedure. So, this is transfection, or gene transfer, these are equally applicable names, and what was found on this occasion was, that one got foci of transformed cells. And these foci, for all practical purposes, looked just like the foci that Howard Temin had gotten years earlier, by infecting monolayers of chicken cells, with Rous Sarcoma Virus. And therefore, that suggested very strongly, that the DNA of the bladder carcinoma, carried within it, an oncogene that was capable of transforming these recipient cells, into which the DNA had been introduced by the transfection procedure. It remained, of course, to actually find that. I'll mention to you in passing, that one does a control experiment here.
If you take normal DNA, you do the exact same experiment you never get foci. So that means, it's not as if all human DNA carries oncogenes in it. The normal DNA doesn't give you foci, the DNA from the bladder carcinoma does give you foci.
And so, about 20 years ago, one actually looked at the normal DNA and the tumor DNA, and one came up with the following, that the bladder carcinoma oncogene, was about, let's say, 6KB long, there was a corresponding, normal proto-oncogene.
It was also 6 kilobasis long. This was a normal DNA, this was extracted by cloning, gene cloning, from the genome of the bladder carcinoma. And having extracted it, one then began to look at how different these two genes were from one another. This transformed cells, this did not transform cells.
If one did restriction enzyme mapping, one found the identical array of restriction enzyme sites. So it was clear that even though these two genes, we can call the normal one a proto-oncogene, these two genes were structurally identical, they couldn't be absolutely identical, because biologically, they were behaving very differently.
And so, when the sequence analyses were done, it was discovered that the difference between the normal proto-oncogene and the oncogene, was a single point mutation, a single-base pair change.
And that single-base pair change created a potent oncogene.
And that single-based pair change, one could show in comparable tumors, was a somatic mutation. Remember, we said somatic mutations are mutations that strike the genomes of cells in our soma, rather than the germ line. That somatic mutation had almost surely had struck one of the epithelial cells lining the bladder of Mr. Jones' urinary bladder. How did this work?
Well, let's go back to our discussion of growth factor receptors, you recall we talked about them last time.
Let's say, here's a growth factor receptor at the cell surface, the growth factor receptor sends signals into the cell, and such a sequence of signals, where you go from a to b, to c to d, is sometimes called a signaling pathway, sometimes it's called a signaling cascade, It's a molecular bucket brigade where a passes signals to b, passes signals to c, to do, and so fourth, and they cross-communicate with one another to process this incoming signal from the growth factor activated receptor.
Now it turned out that the protein product of this, of a normal proto-oncogene, and the bladder carcinoma oncogene, sat right down here, in the signaling cascade, downstream in the signaling cascade of the growth factor receptor.
And the protein product was a very interesting protein, it was a protein, which came to be called RAS, in fact, the original proto-oncogene had previously been associated with a retrovirus. But in this case, it was clear that the activation of the proto-oncogene to the oncogene had nothing to do whatever, with the intervention, by a retrovirus, or by the acquisition of a retrovirus, by a normal proto-oncogene.
So RAS has the normal, the following normal lifestyle.
RAS normally exists in a quiet state, an inactive state, so here's RAS, and while it's in the quiet state it binds GDP, guanosine diphosphate, GDP. What happens, then, is that RAS on occasion, gets an incoming signal from some upstream activator, and you can imagine what the upstream activator is from here.
Keep in mind, I'm only focusing now on, let's say, component c of this signaling cascade, so an upstream activator comes in, and impinges on RAS. And in so doing, it wants to switch RAS from a quiet to an activated state. So what happens, when RAS gets a signal from, an upstream signal from here, RAS will shed its GDP, and will instead, allow a GTP to jump aboard. So now a GTP can jump aboard, and now RAS is in its active state up here. And while it's in its active state, it can emit growth stimulatory signals into the cell, like that. I'm not showing you exactly what it looks like, but it can emit signals. And this is, by the way, called a signal transducing protein, a protein that tranduces signals receives signals from higher up in the cascade, and passes them on lower into the cascade. So it's transducing these signals, and so RAS is put, here I should've capitalized RAS here, so RAS is now in its active state.
It's received upstream signals, it's shed its GDP, its bound GDP, and while it's there, RAS passes signals on further downstream in the pathway. So, if want to relate it to the signaling cascade, and we imagine that RAS is component c of this cascade, it receives signals from b, and then RAS passes signals onto d, it's sitting in-between them, it's an intermediary, it's a member of this molecular bucket-brigade.
Now what happens is that RAS is in this active state for only a period of usually milliseconds, and after it's in a period of this active stage, and it's emitting growth stimulatory signals, downstream, for a period of milliseconds, RAS does something very interesting. It hydrolyzes the GTP, and when it hydrolyzes the GTP into GDP, obviously an inorganic phosphate comes out, RAS switches itself off, i.e. RAS has an intrinsic GTPase activity, GTPase means it can cleave GTP. So it's as if there was a switch here, and I could turn it on, well I'm not going to mess with it since I still haven't figured out how the switches work, but it's as if there was a light switch here that I could switch on, and the lights would be on for a short period of time, and then the switch would automatically shut itself off.
A negative-feedback control to ensure that the period of activation of the RAS protein is only a period of milliseconds, and that the pulse of downstream signals that are released is finite, circumscribed, limited, it's only a quantum of signals that are released. After which occasion, RAS hydrolyzes it's GTP and shuts itself off. It's a nice system, and it actually works because, as I've told you before, we go through ten of the sixteenth cell divisions, with RAS proteins in our cells, and rarely, if we lead virtuous lives and listen to everything I've said in the last lecture, do we ever get cancer. So, what happens when the RAS encoding gene becomes mutated in cancer? That's another one of those questions that I'm really glad I asked, because what happens is that the ability of the GTPase activity to function, is knocked out.
The GTPase can no longer operate, and therefore, the ability of RAS to shut itself off, by cleaving GTP down to GDP, is now compromised, and as a consequence, RAS is trapped for extended periods of time, for minutes and hours, and maybe even days, in this excited signal-emitting configuration, on which occasion, it sends out a continuous flood of signals.
In fact, we now know that the point-mutation, which happens in the gene here, and is seen, by the way, in about 20% of human cancers that have virtually identical point-mutations, those, that point mutation is in the GTPase domain of the RAS protein, that is normally responsible for cleaving GTP into GDP.
So now we can begin to get a very concrete and specific understanding, an insight, into the mechanism by which a point mutation, a somatic mutation, can have a dramatic effect on the ability of a cell to grow. Note, by the way, that if RAS is sending signals constitutively down here, and you may recall that the word constitutive means, at a constant and unrelenting fashion. So the ability of RAS, which I depicted as residing right in here in the signaling cascade, to send out signals unrelentingly down like this, means that the signals up here are no longer so important.
RAS might require a brief stimulus to get into this activated state, but then it can sit around for hours and days, pushing the cell to proliferate. And the subsequent exposure of a cell carrying a RAS oncogene is now gratuitous, it's unnecessary, because this downstream signal emitter has gone wild, and is firing and pushing the cell to grow unrelentingly. Interestingly, that signal is very critically important in pushing cells to move through the G1 phase of their growth and division cycle.
In other words, it's during that phase of the division cycle, that RAS is actually able to send it's signals that perturb cell cycling, and push the cell to move from G1 up to the restriction point, and then into the remaining part of the cell cycle. So now we see how somatic mutations, and we now know about dozens of such genes, can convert proto-oncogenes to oncogenes, without the intervention of any retrovirus.
There are yet another class of genes, which are called, tumor suppressor genes, and these tumor suppressor genes operate in exactly the opposite way as proto-oncogenes and oncogenes.
The proto-oncogenes and oncogenes, from what I've told you, you can imagine are functioning analogously to accelerator pedals on a car, they push the cell to proliferate and, when you have a cancer, the accelerator pedal gets stuck to the floor. In other words, it's no longer well regulated. The tumor suppressor genes work in an opposite fashion, as a breaking system to slow down cell proliferation. And, as such, tumor suppressor genes operate to counteract, and counterbalance, and limit, the growth stimulatory signals that are coming from the RAS gene, and other growth promoting genes. So there's two sides to the coin, indeed as you can imagine from circuit theory, any positive signal must be counter balanced by a negative signal, so that you end up having some kind of physical balance that is compatible with normal, biological function.
So these tumor suppressor genes are normally functioning as break, break linings of a cell, if you will, and that also describes how they become involved in cancer. The mutation that struck the RAS gene caused a hyper-activation of the proto-oncogene.
The proto-oncogene was hyper-activated, and now it began sending out an unrelenting stream of growth-stimulatory signals. As you can imagine, as it is intuitively obvious, in the case of tumor suppressor genes, when they became involved in cancer, what kind of mutations affect them? Inactivating mutations. So there's a very interesting gene, it's called the retinoblastoma gene, and the retinoblastoma gene, the retinoblastoma is a rare eye-tumor of children, it happens about 1/20,000 kids, and the retinoblastoma gene, if you look at the retinoblastoma gene in the genomes of tumor cells, these are the eye cells that form the precursors, the rods and the cones, and other neuronal cells lining the retina. Here's the normal retinoblastoma gene, and it's 190 kilo basis long. So it's a pretty nice size gene, it's not the biggest, it's not the smallest.
If you look at many retinoblastoma tumors, what you find is that there are major portions of the gene that have been just deleted.
Here I'll show you one deletion, here's another deletion, here's a third deletion, sometimes the whole gene is deleted, and cut out. Obviously such deletions are not enhancing the function of the retinoblastoma gene, obviously they're wiping it out. And therefore, we can begin to imagine that the way that the tumor suppressor genes are recruited into the process of forming cancer is through their elimination, rather than through their hyper activation. In fact, as you might also imagine, a cell, which has the following genotype, RB+, RB-, actually grows normally.
Why? Because one of the two alleles has been inactivated, the minus, but the other allele is still, is still active, and still functional, and still able to create an adequately functional break lining. Only when a cell becomes RB- like this, homozygous minus, does it begin to grow uncontrollably, because now it lacks all ability to manufacture the normally required break lining. And this begins, as well, to explain certain kinds of hereditary cancers, because in certain individuals, they inherit a defective allele of the RGB, and therefore, at the moment of conception, they have the following genotype.
Well, you'll say that's all, that's fine, because each of their cells has a wild-type copy of their RB gene, and a mutant, defective copy. And the wild-type copy, as you will correctly say, suffices to template, to orchestrate, to program, normal cell behavior.
Why do they then get retinoblastomas?
Because the surviving wild-type gene, or the gene copy, the wild-type allele, can be lost with a certain, finite probability per cell generation, through chromosomal mistakes, accidents, or through crossing-over.
And so roughly, one in ten to the sixth cells, one has an event, a genetic accident, which causes the accidental loss of the surviving RB allele, leaving, RB wild-type allele, leading now to a genotype that looks like this, homozygous mutant, or homozygous inactive, and now a rare cell in which that has happened, now begins to proliferate uncontrollably because of the absence of the RB break lining, which is normally required to control cell proliferation. By the way, I'll tell you in passing, without describing the gory details, that the RB protein works at the end, at the restriction point. We talked about the restriction point last time, you remember? The RB protein actually prevents the cell from advancing through the restriction point gate, unless it becomes inactivated, and then the cell can sail through into the rest of the cell cycle.
In cells that lack the RB protein, the guardian of the restriction point gate is no longer there, and therefore the gate is held open, and cells can sail all the way through G1, in an unimpeded fashion, without the RB protein standing at the restriction point gate and saying, advance no further, unless and until, certain conditions have been satisfied. And so we can begin to understand that the tumor suppressor gene, the RB gene, is able to act as a quality control, to ensure that cells don't inadvertently, inappropriately, pass through the restriction point gate into the rest of the cell cycle.
And by now, there are 40 or 50 different kinds of tumor suppressor genes that are found to be inactivated through various mechanisms, in human cancers. This was only the progenitor, the harbinger, of those. How many tumors does a child who's born like, this get in his eyes, or her eyes?
Well, it might get two or three or four, in both eyes, but that reflects the fact that there maybe a million or two million cells in each of the retina, which are susceptible to this loss of the surviving wild-type gene copy. Having heard all that, you will ask me, well why don't they get tumors all over the body, since it is the fact that the RB protein regulates the restriction point gate in all cells throughout the body?
So therefore, why isn't the child who's born constitutionally, whose genetic makeup is this, why isn't a child like this sensitive to developing tumors all over his or her body?
In fact, children who are born genetically with this genotype, get retinoblastoma's with high probability early in life, and as teenagers, they often come down with osteosarcomas, which are tumors of the bones. But otherwise, they don't get many kinds of cancer, and the answer to your question is, I haven't the vaguest idea. No one knows why loss of this critical gene that plays a key role in the biology, in the metabolism, of all cells throughout the body, can be lost to yield cancer in the eye and in the bone, whereas when the same gene, which must be lost elsewhere in other tissues in the body are lost, tumors do not arise. And on that puzzling note, I wish you a pleasant day, another happy Halloween, and see you on, oh yes, tomorrow is election day.
Don't forget to vote. And remember what the mayor of Boston once said, vote early and often.
Free Downloads
Free Streaming
Video
- iTunes U (MP4 - 152MB)
- Internet Archive (MP4 - 152MB)
Audio
- iTunes U (MP3 - 12MB)
Caption
- English-US (SRT)