Flash and JavaScript are required for this feature.
Download the video from iTunes U or the Internet Archive.
Topics covered: Immunology 1
Instructors: Prof. Robert A. Weinberg
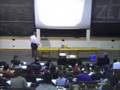
Lecture 22: Immunology 1
Today, we're talking about immunology as you may recall.
Let's roll. Yes, let's roll.
And by the way, admire your little finger, because most of the planet isn't as gifted as you are.
Not everyone was guaranteed so many brains as you and I, seriously, have been fortunate enough to have been granted.
Let me just finish up what we were talking about last time, which was the cell cycle.
You may recall that we talked about oncogenes. We talked about Ras and the Ras oncoprotein, which sends out mytogenic signals.
And, what it does, just to summarize what we were talking about is it pushes cells from the beginning of the G1 phase of the cell cycle up to a decision point in the life of the cell.
It's called the restriction point. And here, just by way of review, the cell has to decide whether or not it's going to commit itself, essentially irreversibly, to go through the rest of the cell cycle, or whether it'll stay in G1, and may even retreat from the cell cycle into G0. And Ras pushes this decision forward.
The retinoblastoma protein, which we talked about the last time, stands as the guardian of the gate right here, the RB protein.
And, the retinoblastoma protein holds this gate shut unless and until certain preconditions have been satisfied, on which occasion the retinoblastoma protein opens up the restriction point gate, and allows the cell to go through the rest of the cell cycle. And now, interviewed from this perspective, we can begin to understand how hyperactivity of Ras, and how the inactivation of this RB, this retinoblastoma, tumor suppressor protein have such disruptive destabilizing effects on the proliferative controls of the cell.
Keep in mind, this is a negative actor on cell proliferation.
It's a tumor suppressor gene which must be inactivated in many cancers.
This is a proto-oncogene, or an oncogene, which must become hyperactivated. Now, I want to move from that into the topic of today, and that's the whole issue of immunity. And, much of our immunity comes from understanding the way we deal with what viral infections.
The fact is, just to cite one arbitrary viral infection, relatively few people die from viral infections these days because we can become immunized against them. The first immunizations already began in the late 18th century, believe it or not, when a physician in England called Edward Jenner first noticed that women who had worked as milkmaids milking cows, and who got a disease called cowpox from milking the cows seemed to be immune to the disease of smallpox which was by that time realized to be spreading in epidemic, so a highly infectious agent, and one which actually killed quite a few people. And Jenner intuited correctly, in retrospect, that the experience of these milkmaids and their exposure to cowpox exposure somehow protected them, gave them indeed lifelong protection from subsequent smallpox infection.
Subsequently to that, the sores from the cowpox infection were scraped, and the exidate, the fluid, was scratched into wounds of people in order to immunize them. And so, immunization them. And so, immunization already began in the 1790's, taking a sore from the skin of a cowpox infected patient, injecting that into the skin of somebody who required immunization, and as a consequence, hoping that this would confer in them lifelong protection.
In some cases, actually the individuals who were infected in that way actually came down with smallpox or some virulent form of this cowpox, but in most other cases these individuals actually acquired a lifelong immunity.
In fact, the very word vaccine, which was used already at the time, comes from the Latin word vaccinus which means a cow.
And, in the north side of Cambridge Common there's the Benjamin Waterhouse which is still there. He was the first physician to introduce smallpox vaccination into this country already in the end of the 18th century. If we fast forward to a situation like poliovirus, we have situations in this country where in the 1930's-1940's, there were epidemics of poliovirus.
If one began to examine who was susceptible and who wasn't, it was clear that children who were, for example, born and raised in middle and upper-middle class houses were very susceptible for much of their lives. For example, in southern California there were dramatic examples, whereas children who grew up across the border in, let's say, Tijuana, Mexico, rarely came down with paralytic polio.
Poliovirus, as one soon learned, was a virus which infects not only the gastrointestinal tract, and creates a form of mild diarrhea, but it may be one out of 100 persons the virus escapes from the GI tract, from the gastrointestinal tract, invades into the central nervous system, and actually creates debilitating paralysis, most of which is not healed. And some people have lifelong paralysis. Other individuals whose paralytic paralysis goes away, actually when they grow older, 30, 40, 50 years later, they begin once again to experience the paralytic symptoms that arose as a consequence of their childhood infection. And at this time, one began to try to figure out why children living in Tijuana, Mexico rarely came down with poliovirus infections, whereas those who grew up up north like, say, in southern California did indeed do so.
And one came to the conclusion that the children growing up in Tijuana, Mexico were frequently exposed very early in their life to contaminated water, sewage contaminated water, and they as a consequence acquired a lifelong immunity without getting sick, whereas children who grew up in rather sterile conditions further north never had any exposure to the virus. And when it hit them as young adults or as teenagers, it created devastating effects. And this indicates, once again, that somehow one's exposure to an infectious agent, historical exposure has a dramatic effect on one's susceptibility to a virus. And in the case of poliovirus, we're dealing here with an agent which is much simpler than the retroviruses we talked about last time like RSV.
Poliovirus also has a single stranded RNA genome, and that's encapsidated in a proteinaceous coat, which is made up only of viral proteins, so it's very simple single stranded RNA proteinaceous coat. The single stranded RNA is actually polyadenylated at the three prime end. So, it can serve as a messenger RNA. It's of the same polarity that has a plus polarity, which means that it can be translated immediately. I'm distinguishing that from the other flavor of single stranded RNA which could be of the complementary strand, which could exist because one could make double stranded RNA which obviously cannot be translated.
So, this can serve as a messenger RNA, and just as an aside, the way that poliovirus replicates is totally different from that of retroviruses. What happens is that poliovirus makes its own polymerase.
You go from single stranded RNA to double stranded RNA, i.e. the complementary copy is made, and that in turn is used as a template for making more single stranded RNA, progeny RNA.
And note here that there's no DNA at all involved. In fact, poliovirus can grow inside a cell that has been deprived of its nucleus. Moreover, if one stops nuclear DNA dependent transcription, that has no effect on this.
But you'll notice correctly that these kind of steps here involved RNA dependent RNA polymerases. And in the normal physiology of a cell, that such a polymerase, such an enzyme never operates.
And therefore, poliovirus must make among its other proteins an RNA dependent RNA polymerase that can mediate these steps: making a complementary copy of this strand, to get the complementary strand, and then making progeny plus strand RNAs.
Again, it has a very simple capsid of several viral proteins which wrap it up. Now, if one wants to assess the potency of poliovirus RNA, one can grow it in a Petri dish. One way of figuring out how much poliovirus RNA is in a solution is to make a monolayer of cells like this, and then take various dilutions of a virus stalk.
And when one talks about a virus stalk, one talks about a solution of virus particles. One can't really see them.
And even if one could see them under the electron microscope, one wouldn't really know what fraction of the ones you were seeing were actually biologically competent. But most interestingly, you can take a solution of poliovirus particles, place it on a monolayer of cells here, which can be infected by poliovirus. In contrast to what I told you last time about RSV, where an infected cell can tolerate the continued presence of a viral infection without dying, poliovirus is a highly cytopathic virus, and by that I mean it replicates inside cells and it kills them during the course of replicating inside these cells. So, here what one has is just a poliovirus will infect a cell here, and then it will begin to spread from that cell to neighboring cells in the surrounds.
And in so doing it will create, it will erode a hole right here in the monolayer whose presence, a so-called plaque, signifies the fact that there was an initially infecting virus particle there that spreads centrifugally, that spread outward, from the initially infected cell. In detail, if you want to do this experiment really nicely, what you do after you infect the initial monolayer of cells, which I show here in section, is you put on a layer of agarose, or something, above the infected monolayer, and that ensures that if there's any viral spread, it will be from cell to cell spread in a certain neighborhood rather than virus particles getting into solution and swimming around all over the plate and affecting cells helter-skelter.
So here, we might want to infect an initial cell right here in the monolayer, and now this agarose will confine the subsequent spread of progeny virus to adjacent cells in the area, again eroding, ultimately, a hole here which we call a plaque.
And if we count the number of plaques, that will tell us how many biologically active virus particles there were in the initial solution that was previously applied to that monolayer culture of the susceptible cells. One could use such monolayer cultures, in fact, to propagate poliovirus. And one can take the poliovirus coming out of those monolayer cultures, and actually use them to inject into human beings in order to vaccinate them to confer on them immunity. But if you do that with wild type poliovirus, then what will happen is that you may inadvertently give that person whom you're attempting to immunize a nasty poliovirus infection which could paralyze them, might even kill them.
When I was growing up, poliovirus infections were much dreaded because we knew of people who were being kept alive in iron lungs that breathe for them because their autonomic nervous system had been destroyed by a poliovirus infection.
So, what happens is therefore if one wishes to immunize somebody, if one wishes to vaccinate them against poliovirus, one needs to inject them with an attenuated virus, that is, a virus whose ability to create disease, whose pathogenic abilities, pathogenic, remember, refers to the ability to create disease. You want to use an attenuated virus, which might be able to go into the body. It might be able to evoke immunity, but it will not create a major disease response.
And this was dealt with in two different ways.
Jonas Salk, one of the pioneers of creating a poliovirus vaccine took the poliovirus particles that have been produced by culturing them on monolayers of monkey kidney cells, and he treated the poliovirus briefly with a little bit of formaldehyde.
And formaldehyde, as you may know, reacts with the amine groups of the ribonucleosides, of the purines and pyrimidines, and therefore kills the virus. And that kill virus, having been treated briefly with the formaldehyde, the virus particle is still essentially intact, was then injected into individuals.
Alternatively, his deadly rival, because they two hated each other's guts, Sabin decided on another strategy for making a vaccine stalk.
And that is, he took poliovirus which had been passage from one cell culture to the next over extended periods of time, over several years. So, it had been passage in vitro.
When we say in vitro, in this context we mean poliovirus had been taken from one plate, put into another plate of cells, taken to another plate of cells. In vitro here implies in culture rather than the other alternative. In vivo means that the virus is being passaged in a living organism. And as it turns out, when you passage poliovirus in vitro from passing it just from one culture of cells to the next, infecting one after another in a serial or sequential fashion, then the virus is selected for its ability to proliferate in the cultured cells in vitro.
But it gradually loses its ability to create disease in vivo in that there is no Darwinian selection to favor its disease causing ability.
And consequently, virus that Saben used wasn't inactivated with formaldehyde. It was a simply attenuated because of extensive in vitro propagation. And when he injected that into young people, very rarely did they ever come down with poliovirus infection, and the virus was able to replicate to some extent in their bodies without causing disease, and to create lifelong immunity.
I.e. such an individual was protected from subsequent poliovirus infection ever again. And, as it turned out, this was a very nice way of protecting because it also turned out the monkey kidney cells that were used to propagate the virus also contained certain monkey viruses. For example, it came out after years that there was a DNA tumor virus.
We've been talking about RNA tumor viruses until now, but there's a DNA tumor virus called SV40, which is a very potent tumor virus in hamsters, and mice, and rats.
And it turns out that SV40 lingered. It lurked in monkey kidney cell cultures. And, nobody knew about it.
Whenever you put poliovirus into many of these cultures, not only did poliovirus replicate, but all of a sudden so did SV40.
And therefore, many of the stalks of poliovirus that were used to inject people like myself, I lived around the corner in Pittsburgh from Jonas Salk, and the children in the Pittsburgh public schools were his first guinea pigs. Many of us were exposed to poliovirus stalk which actually had more SV40 particles in it than poliovirus particles because of this inadvertent contamination.
There probably were between 30 and 40 million people who were immunized with poliovirus and inadvertently with SV40 virus.
And one is that epidemiology in the subsequent years to figure out whether that had led to any increased rate of cancer because it could well have. There could have been an epidemic of cancer in this country, millions of people affected because of this unknown, and at the time almost unknowable contaminating virus. As it turns out, people who were infected with SV40 inadvertently have no higher rates of cancer than anybody else even though this virus is a very potently tumorogenic virus like RSV, but doing so in this case in rodent cells and ostensibly not in humans. So, public health measures can sometimes have unforeseen side effects or consequences that nobody anticipates ahead of time. Now, getting back to the whole strategy of poliovirus infection, this raises the issue of what it was in the poliovirus that was able to evoke the subsequent lifelong immunity. And in telling you that, I mentioned the following.
You can make antiserum or you can make serum from an individual who's been immunized with poliovirus. And, recollect, we mentioned this before, the way to make serum is to allow blood to clot.
The red cells and the platelets aggregate in the bottom, and what remains on top is simply serum. You can spin out all the residual cells and so all you get is sort of a straw colored fluid.
And that serum from an individual who's been immunized with poliovirus stalk can actually be used to, you can add it to the poliovirus stalk prior to adding these virus particles to the Petri dish.
So, here's the Petri dish as before, and what you find is that if you add serum from an individual who's been immunized to a solution of poliovirus particles, and then you take that mixture and put them on the plate, you no longer get any of these plaques that I referred to before.
And that indicates that within the serum, there is some kind of factor which is neutralizing the infectivity of the polio virus particles, i.e. the poliovirus particles are in some way prevented from creating an infection, and their plaque forming ability, their ability corrode these holes in the monolayers of subsequently infected cultures is now compromised.
And such activity suggests that such an individual has actually antiserum, i.e. some kind of reactivity which prevents the poliovirus particle from doing its thing. In fact, I can tell you that such antiserum or such neutralizing antiserum can be achieved in a number of ways. One way to do it is just to take the protein capsid, the outer coat of the poliovirus particle that I described before and inject that into an individual.
And that will on its own already evoke a measure of antiviral immunity. It will evoke a concentration of antiserum in the blood of a patient. Or, if you want to do it in a very much more modern way, you can sequence the proteins of the poliovirus and determine the amino acid sequence.
And when you do that, you can synthesize through organic synthesis oligopeptides of 10 or 20 amino acids long.
And these oligopeptides reflect different parts of the poliovirus coat protein. So, if here's the sequence of one of the capsid proteins, recall that capsid refers to the coat that's shielding or protecting in this case poliovirus, the single stranded viral RNA genome. So, if here's one of the capsid proteins, what you can do is to figure out the amino acid sequence of this segment of the capsid protein, and then rather than cutting it out from the capsid protein, you just synthesize it by organic synthesis, ten or twenty amino acid residues long. And when you inject that oligopeptides into an individual, that may also evoke some kind of neutralizing antibody response. In fact, as you might correctly imagine, if we can look in more detail at the capsid structure, I'm just going to draw it in a very primitive way here, it's obviously much more complicated. But if this is the capsid here, it has an inside and an outside. And the antibodies that you make against an oligopeptide that is sticking out here on the outside will be neutralizing, i.e. the antiserum will be able to recognize the outside of the virus particle and somehow inactivate it.
But if you were to use an oligopeptide antigen from over here, which might be a part of the capsid protein that is deep inside, well even though you might have a good reactivity with oligopeptide it would not be neutralizing. And that suggests the notion that whatever is present in the antiserum is recognizing some external portion of this protein which is sticking out. And that external portion is called an antigen. So, there's a specific chemical structure which is being recognized by the antiserum, and we call that an antigen. In principal, there are dozens of distinct antigens on the surface of a poliovirus particle, each of which one can, in principal, make an immunizing antibody against.
Each of those can therefore be considered to be an antigen.
And, how can we imagine this antiserum as actually successfully neutralizing the virus particle? And here, this depended on actually the biochemical characterization of antisera because one soon came to learn that within antisera are what we call antibodies.
Or, keep in mind that in biology you never should ever use a simple Anglo-Saxon word if you can use a much more complicated Greek or Latin one. So, we can always call these immunoglobulins.
You see the word right there. Immunoglobulin represents an antibody molecule, and it turns out that much of the protein in the soluble fraction of our serum consists of such antibody molecules. And these antibody molecules have a very interesting structure which I'd like to dwell upon momentarily.
But before I do that, I'd just want to anticipate what I'm about to say by indicating that we now realize that an antibody molecule can recognize an antigen on the surface of the virus particle, and the antibody molecule can actually physically bind to this antigen. So, we have an antigen antibody complex, and that binding of the antibody molecule to the surface of the virus particle is what results functionally in the neutralization of its infectivity. Or to put it another way, given the fact that this particular capsid protein might be repeated, let's say, 60 times around a spherical surface of a virus particle, there might be, in fact, 60 different antibody molecules recognizing this repeated antigen which occurs on each of these capsid proteins. And therefore, you can imagine in a very approximate way that if you have an effective neutralizing antibody, it's coating the surface of the virus particle of antibody molecules, and that clearly obstructs the ability of the virus particle to initiate a subsequent round of infection. Here's what a single antibody molecule looks like, and it has some critically important features. First of all, there is a specific region of the antibody that recognizes the antigen. In this case, I'm using as the example the antigen an oligopeptide on the surface of the poliovirus particle. And that is located in this part of the antibody molecule.
Note, by the way, the antibody molecule is a heterotetramer.
It has two light chains, two small chains here on the right, and two heavy chains. Note that the cysteine disulphide bonds are holding the entire assembly together. So here, one is not relying on hydrogen bonds to hold together this heterotetramer.
And note the following, that there are portions of the antibody molecule which may be specifically varied from one antibody molecule to another over here, and other portions which are standard operating hardware. And let me elaborate on that for a moment. If you look in the serum of an individual and you try to analyze the chemical structure of the antibody molecules in their serum, you find that for a given type of antibody molecule like this, all the antibody molecules share this constant here: light purple domain. They all have that in common.
They all have in common also the light blue domains.
But if you were able, and we'll discuss this in a moment how you could do so, if you were able to analyze this other portion, you would find that when you look at one antibody molecule, it has a certain amino acid sequence here, which is involved in antigen recognition, and that this amino acid sequence on the light and heavy chain varies from one heterotetramer to another heterotetramer. And again, I'm going to reinforce that. But I want to indicate that there are, within the human antiserum, millions of structurally distinct antibody molecules.
They share in common the structure down here up to this point.
And thereafter, each of them has its own idiosyncratic, its own unusual, its own particular private antigen recognition site, which stems from a region or a pocket of the protein which is involved in antigen recognition and has variable amino acid sequences.
Here, you see the way that an x-ray crystallographer would actually visualize this molecule, and you begin to realize that the schematic that I showed you before, in fact, is really doing a little bit of violence to the real amino acid sequence, to the real three dimensional structure, excuse me.
Now, note something else that is implicit in what I've just said but I haven't said it explicitly, there are actually two antigen recognition sites, one here and one here.
So, this antibody is in that sense bivalent. And if there were, for example, two poliovirus particles, one over here and one over here, you could imagine in principal, although I'm not drawing things to scale, that this antibody molecule could actually use one of its antigen recognition sites to bind over here to one poliovirus particle, and this to bind to another poliovirus particle. Now, I've told you that the antigen is recognized by this antibody is an oligopeptide, which constitutes a distinct chemical structure. How many different oligopeptides of ten amino acids long are there in the mathematical universe?
How many conceivable ones are there?
How many amino acids are there? 20? So what's the number? I heard you all say 1020, right? So, that's an awful lot.
That's more than you can shake a stick at. So that means that there are, in principal, essentially an infinite number of oligopeptides of ten amino acids long, and each one of those could, in principal constitute a distinct antigen that could be recognized by the antibody molecule. Note that what's happening here is that a protein antigen such as one of these oligopeptides is being recognized by another protein, which is the antibody molecule.
And therefore, we begin to imagine there's some kind of lock and key complementarity where somehow the amino acid sequence here recognizes and binds a complementary fashion to the antigen that is being recognized, and there's a physical association which allows the antibody molecule then to attach tightly to the antigen bearing poliovirus particle.
And, by the way, to put it another way, it could be that some of these poliovirus capsid proteins have not yet been assembled in a virus particle. And even if they aren't, in principal a free protein, a capsid protein of poliovirus, could also be recognized by an antibody molecule and be bound by the antibody molecule. Now, this creates several major conceptual problems. How on earth can the body make antibody molecules that are able to recognize a poliovirus particle?
Well, you'll say that's easy. Clearly, in our genes there must be some kind of nucleotide sequence which has up here the ability encode an antigen-binding site. Note, by the way, the antigen-binding site's actually composed of two different proteins.
Here's part of the antigen-binding site. Here's another part of the antigen-binding site. So, it must be on two different genes. But, these two different genes create an antigen-binding site which can recognize poliovirus. Bind the poliovirus antigen and neutralize it. Well, that's well and good.
That's perfectly reasonable, but let me tell you what's unreasonable about that. Each of us during his or her lifetime are going to be infected by literally hundreds of viruses.
You get cold viruses almost every two, three, four, five times a year. By the time you get old, you actually have acquired immunity to many of these, but during the course of one's lifetime, the immune system has been able to generate antiviral immunity and eliminate virtually all the viral infections you ever got. How many people do you know who have actually died of a viral infection? And yet each one of these viruses is in principal able to replicate throughout your body and kill you, and they never do? So that means that the immune system is extremely effective in developing an antibody or antibodies which could neutralize infecting virus particles. You might have the cold for a day, a week, or two weeks, but eventually the immune system develops enough antibodies and other strategies to eliminate the viral infections from your body. I remember when my grandfather was 94 years old and he got a cold. And he hadn't had a cold for 20 years because by the age of 75, he had been exposed to almost every virus imaginable. And so, when he got a cold at the age of 94, his brain was still working, he'd forgotten how to blow his nose. It just hadn't happened to him. He didn't know what it was like to have a cold anymore. So, it just goes to show you that the immune system can be very versatile and very successful.
But this creates a major conceptual problem. How do our genes know how to create antigen-binding sites in our antibodies that recognize all of the infectious viruses that are going to attack us during a lifetime. Do we have a gene for neutralizing smallpox? Do we have a gene for neutralizing poliovirus and adenovirus, which gives us bad head colds. And, rabies virus and vesicular stomatitis virus, and all other kinds of viruses that we are going to experience in a lifetime. Well, possibly we do have a gene for each one of those. But, then I'm beginning to raise some other questions if you believe that, which you shouldn't. First of all, there are many different distinct oligopeptide antigens on the surface of the poliovirus particle. If you look at the antibodies that had been developed against a poliovirus capsid protein in an immunized individual, they have one antibody that recognizes one part of these capsid protein, and another antibody that recognized another part. So, here's the surface of the capsid protein blown up now, and we can imagine there are different oligopeptide epitopes on different parts of the protein.
And they're all ten amino acids long, for example. The fact is, in individuals who have anti-poliovirus antibody, you can find that there's an antibody that recognizes this antigen, and one that recognizes this antigen, and another that recognizes this antigen. So, the number of distinct antigens which the immune system has succeeded in making antibodies against, vastly exceeds the number of infectious agents we're going to experience in a lifetime. And, that begins to undermine your confidence in the notion that when we're born, we already possess the genes to recognize different infectious agents, and to construct neutralizing antibodies against them.
There's also another flaw in this whole notion that we inherit a set of genes that enable us from the get-go to make specific antibodies. And that flaw comes from the following notion. Evolution cannot anticipate which infectious agents each one of us is going to experience in a lifetime.
Let's say that some of us experience a totally new kind of a viral infection which had never been experienced by our ancestors.
If the way of neutralizing that virus, and defending us against that infection depended on using an antibody molecule whose sequence was encoded in our germ line in the genes we inherited at birth, then we might be in bad shape if that virus was a novel virus to which the human race had never been exposed.
And in arguing that, I'm telling you that it's impossible to imagine a situation where our germ line, the set of genes we are born with that we start life with, already contains the information for making each one of the different kinds of antibodies that are in our antiserum. How many different kinds of antibodies are in our antiserum? Well now, the whole notion becomes even more stark because there's probably millions, maybe even ten or 100 million distinct antibody molecules floating around in our blood. Each one in this case has the same constant region down here. You see the constant region, and each one of these 100 million distinct antibody species has its own antigen-combining site. Now, if we want to pursue that further, we have to begin to imagine how the immune system can make so many different antibody molecules. This itself is a major conceptual challenge. And now we have to go and know a little bit of cell biology because if we look at the immune system, and there are cells forming in the immune system, we find a set of cells that are called B cells or a subset of B cells that are called plasma cells. And the plasma cells, and there's a whole bunch of different plasma cells.
So the plasma cells are floating around in the circulation, and the plasma cells are able to secrete antibody molecules into the serum. And they are abundant. They're around by the billions inside our body. And these plasma cells are constantly secreting antibody molecules into the serum around them into the plasma around them. And these are the antibody molecules I've been talking about here, the antibody molecules that are capable of neutralizing poliovirus particles.
So, this now creates another conceptual question.
Let's imagine for the sake of argument that in our blood stream there are 100 million distinct antigen species floating around.
And I don't mean 100 million molecules. I mean there's a million molecules, and each one of these species has a different antigen-binding site. So, there may be many antigen-binding sites. There may be many antibody molecules. There may be million of this kind of antibody that recognize this antigen. There may be millions of this antibody recognizing this antigen, millions of this antibody recognizing this antigen on the surface of poliovirus.
And I'm saying there could be a million distinct species of antibodies, each species being defined by the antigen that recognizes and by the structure of its antigen-recognizing site, its antigen-binding site. In other words, to repeat myself, what distinguishes one species of antibodies from another is the amino acid sequence, the structure of this particular region of the antibody molecule that makes one species of antibody different from the other.
And with all that in mind, we have two alternative scenarios.
Let's again say there's a million different species of antibodies being secreted into the antiserum at any one point in time.
This cell could make all million. This cell could make the whole million species. This cell could, and this cell could. In other words, each one of these B cells could be multitalented, able to simultaneously make a million different kinds of antibody molecules. The opposite model is as follows, and that is that this B cell makes one species of antibody. We'll call it antibody A, and this species makes another species of antibody molecule.
So, this B cell makes antibody A. This B cell makes antibody B. This B cell makes antibody C. And keep in mind that when I'm saying antibody A, B, and C, I mean A has a certain antigen-binding site. Be has another antigen binding site.
C has yet another antigen binding site. And, we can distinguish between these two alternative mechanistic models by looking at the disease called multiple myeloma. And what you have in multiple myeloma is the following. All of a sudden, the blood stream becomes full of much greatly elevated levels of antibody molecules. They're present in the blood stream.
Now, normally, if you look at all the antibody molecules in the blood stream and you were to separate them by some kind of electrophoretic technique which distinguished them on the basis of subtle differences in the amino acid sequence, and let's not worry about exactly how you do that.
But we've talked about a million different antibody species.
They're chemically slightly different from one another by virtue of the antigen-recognizing site. And if you could separate them by some kind of system that separated them on the basis of their charge, you'd find a whole spectrum of different antibody molecules, a million in this large, heterogeneous mixture of antibody molecules, each species found somewhere or another in this very broad distribution of 100 million distinct species which are all mixed together in the antiserum. If you look at the serum or the plasma of a patient suffering from multiple myeloma, what you find is the following, that one species of antibody molecules dominates over all the other ones. And so now, whereas that single species might only be on average one millionth of the total antibody complement in the blood. In those suffering from myeloma, or sometimes it's called multiple myeloma, now one single antibody species may represent 60, 70, or 80% of all the antibody molecules in the blood.
And clearly something has gone very wrong. And if you look at what happened in the case of an individual of multiple myeloma, if we look at that diagram up there, what you see now is that instead of there being many different B cells that are equivalently represented in the blood stream, now one of the B cell types has begun to multiple uncontrollably, and this vasicular B cell type, which I indicate up there, which happens to be specifically able to make antibody C in this model, now this particular species of B cells is now a predominant constituent of the population of B cells in the blood. So just to reiterate, in the normal blood, there are a million different sub-populations of B cells. But in these individuals, one of the sub-populations of B cells has expanded. It's created a monoclonal growth.
Keep in mind monoclonal refers to the fact that all the cells in this tumor, and there is a tumor, descend from the same ancestor.
So, it's a monoclonal growth. It's a kind of cancer.
It's a kind of blood cancer. And all the cells in this population make the identical antibody molecule, which explains this very homogeneous peak here riding above the very heterogeneous mixture of antibodies, the background heterogeneity that normally characterizes normal serum.
And what that clearly indicates is that each different B cell or plasma cell as you will like to call it in our normal serum is specialized to make its own particular kind of antibody. It's not that a single B cell can make a million different antibodies. Each B cell makes a very specific kind of antibody. And when I say a specific antibody or a specific antibody species, again I'm referring to an antibody that has a specific antigen-binding site.
Or to put it another way, all of the antibody molecules coming out of a single B cell are identical with one another.
That, then, raises the question of how the B cell learns how to make that antibody molecule and not other antibody molecules.
There's yet another puzzle we have to answer, and that's the following.
If you look at the serum response of an individual to a viral infection, it looks like this. And here, let's look at what's present in the ordinate, and it's on a log scale as you can see here, and what's present on the abscissa. If one is initially exposed to an antigen and develops an immunity, then here is the wave of antibody production and the concentration of antibody against, let's say, poliovirus that is present in the blood of a recently infected individual. They're exposed to the antigen, or in this case the poliovirus capsid protein.
In the days and weeks that follow, they develop a significant level of antibody which is used to neutralize, in this case the infected poliovirus particles. And then once the poliovirus is cleared from the system, i.e. once all the infectious particles have been neutralized, then the antibody molecules go down almost to zero, and there's virtually no antibody against poliovirus left around.
But look what happens when that individual is re-exposed to poliovirus years later. All of a sudden, now he or she mounts a massive antibody response vastly higher than the initial one.
Well, you say it's only twofold higher than this.
But let's look at the ordinate. This is a logarithmic plot. So, on this plot, the secondary antibody response is maybe 100 or 200 times more vigorous, much higher antibody production.
Let's think about this time years later when that person is exposed to a second virus. Let's say that person is exposed subsequently to cold virus, adenovirus. Does the exposure to poliovirus here in the blue curve help that person develop an adenovirus antibody response? The answer is absolutely not.
There's no cross-immunity. And so, when that individual years later becomes exposed to adenovirus, there's once again the same kind of initial response that happened as a consequence of previously being exposed to poliovirus. It's a rather weak response.
It's effective enough in developing the initial virus eliminating ability, but it's not very strong. What is this telling us? I'm glad I asked that question. It's telling us that that immune system is able to remember over a period of months, years, and decades that a previous exposure to this antigen has occurred because here the immune system in this second red curve knows, somehow remembers, that there was an exposure years earlier. And not only does it remember and respond rapidly, but it responds much more vigorously.
And here now, we begin to recognize some of the outlines of immunity because the immune system remembers this earlier exposure, and when it's provoked a second time, through an accidental and inadvertent exposure to poliovirus on a second occasion, now it really goes to town. And now it creates antisera which is much more vigorous than it was during the first exposure.
If the first exposure had never occurred, there wouldn't be such a vigorous response. Look at this one over here.
So now, we begin to realize two mysteries that we have to explain next time in our further discussion of the immune system.
First of all, how do B cells figure out how to make so many different kinds of antibody molecules. And secondly, how does the immune system remember from one decade to the next, that exposure to a previous antigen has occurred which merits a vigorous response.
See you then on Friday.
Free Downloads
Free Streaming
Video
- iTunes U (MP4 - 137MB)
- Internet Archive (MP4 - 137MB)
Audio
- iTunes U (MP3 - 11MB)
Caption
- English-US (SRT)