This resource is available as audio only.
Flash and JavaScript are required for this feature.
Download the track from iTunes U or the Internet Archive.
Description: This lecture discusses foraging behaviors.
Instructor: Gerald E. Schneider
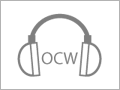
Lec16: Foraging behaviors
The following content is provided under a Creative Commons license. Your support will help MIT OpenCourseWare continue to offer high quality educational resources for free. To make a donation or view additional materials from hundreds of MIT courses, visit MIT OpenCourseWare at ocw.mit.edu.
PROFESSOR: OK. So I want to talk a little bit about foraging behavior, and then that will be followed by some things on antI-predator behavior, and those will be the last topics before the midterm.
We will finish antI-predator behavior next Friday, but it should leave time-- because we'll start that on Wednesday-- we should have time for some reviewing, since the exam is on Monday, not next Monday but the following Monday.
OK. Now this first question you should be able to answer by now, because it's come up before. Does the level of foraging or hunting depend on the amount of hunger? Is it independent of hunger?
And the reason I raise the question again is because in the reading by Scott, he paints a somewhat different picture than the one we learned about from Konrad Lorenz and Leyhausen's work with cats and John Flynn's work with cat brain stimulation.
So what's the simple answer? Is it dependent on hunger? Or not? In fact, in some of the videos I show, occasionally you'll hear mentioned that people just assume that if they're not hungry they won't hunt.
Well, you'll see. We're going to see a video next week where you'll get an example of that that contradicts some of the ecological studies that we know about. So Scott doesn't make it clear that some innate foraging drives are separate from hunger. But remember we discussed this before.
But Scott does introduce one more thing, this. On page 120 he talks about the role of social cues and of learning.
So my question here is, why might an animal eat more when feeding with a group of his conspecifics than it would if it was feeding alone? In fact, sometimes a monkey in a group, he'll finish feeding alone, but then if suddenly he's with a group, he will start eating more if they're all eating.
So why-- what do you think is behind that? Why would that evolve? And when I ask you question like that, why it would evolve, what I want you to think about is what is the benefit for the individual? What's the selfish reason why an animal would do that? He ate to satiation. He shouldn't be hungry, so why would he now start eating?
Did you read it? Because Scott does suggest the reason in the reading.
If all the other animals-- there's a limited amount of food there, and if they're all eating it, there's not going to be anything left. And animals need to maximize their food intake to survive, so an animal will eat more in a social group for just that selfish reason. Eat it before it's all gone. And that's why we think that tendency evolved.
It's also sometimes just called social facilitation by social psychologists. But here in animal behavior, you can call it social facilitation, but there is a specific reason why that should evolve as innate behavior.
Let's go to the next topic here, on conditioned taste aversion. It's also called the poison bait effect. And Scott calls it a form of classical conditioning. And in fact there's a box in the book there, on page 122, where he goes through that.
And it was discovered by John Garcia in California. It has properties that do not fit the descriptions of classical conditioning, and this is why.
For one thing, it's something the animal learns in one trial. And there are examples of other one-trial learning. Like a rat stepping down from a platform onto an electrified grid. He will learn in one trial not to step down.
But most things take longer to learn. At least with positive reinforcement that's true. In this case it's not only just one-trial learning, but this so-called unconditioned response where the animal gets sick and feels nauseated as a result of eating. A lot of times that sickness doesn't occur immediately. It takes a little while, and yet they still learn to avoid that food.
And the third thing is that the memory is much more long lasting than you find in classical conditioning. Humans that have experienced this, and many of us have, it can last for years.
For example, when I was a kid, I was probably on the verge of getting the stomach flu but didn't know it. But we were roasting marshmallows. And often we not only browned them, we burned them a little bit. And I ate a partially burnt marshmallow, got sick afterwards or felt terribly sick, and I couldn't stand the sight of that kind of marshmallow for 20 years. A very clear poison bait effect.
It didn't matter whether the illness was really caused by the marshmallow, because other people didn't get sick from it. I think I was getting sick anyway. How many people here have experienced that kind of effect in their lives? About a third of you.
Does anybody want to tell us? Give us an example? They might like to hear it. Come on, one of you tell us what you experienced.
AUDIENCE: Me?
PROFESSOR: Yeah.
AUDIENCE: [INAUDIBLE].
PROFESSOR: What did you eat?
AUDIENCE: [INAUDIBLE].
PROFESSOR: Well, that's OK.
AUDIENCE: It was like ground-up meat and it had, like, fillers in it. And I just decided it really grossed me out, and I felt nauseous--
PROFESSOR: You felt nauseated afterwards. And it's mainly what causes the effect. And so you-- how did it affect you later? You wouldn't eat anything like it. For how long? Or is it still there?
AUDIENCE: Oh, I got over it.
PROFESSOR: Well, this happened to me when I was about, I would say, seven years old. And I was certainly probably 30 years old before I could eat a marshmallow like that.
All right, so it's not really-- what is it, then, if it's not classical conditioning? Scott has written that conditioned taste aversion is, of course, a form of classical conditioning. Why would he say that?
The reason is, first of all, many learning theorists have lumped all learning into just a few categories. And many textbooks on learning are organized that way. The few major sections, classical conditioning, instrumental conditioning. And now they usually have a few other types, too.
And also many people, including many scientists, have believed, as you know from the class before, that almost every behavior is learned, especially here in America. Lawrence calls this form of learning an innate disposition for learning. The innate schoolmarm that shows us evidence for a built-in program for a particular kind of learning that it's very easy to understand why that would be adapted.
It's been used to control predation of livestock. If you are having sheep being killed by wolves, for example-- it usually has to be a particular wolf pack, so they're all going to eat some-- you give them a sheep, a lamb or something that's been tainted, or calves, or the kind of thing that they're eating. And they will avoid it for quite some time. It's somewhat variable in how effective this is, but of course just how many animals actually eat it and get sick from it will vary too.
Now it's related to another form of avoiding food we call neophobia. Animals tend to avoid new things. So you set a trap for a mouse or a rat in your house. If it's not something really familiar to the animal, they will be very, very cautious. It needs to be something that they really love and has a strong odor. Certain kinds of cheese have that effect and--
[CELL PHONE RINGS]
Sorry. Well, I hope that will wait.
OK. So why would they avoid something novel? Well, it's not as likely to be safe. They know what's safe. They've had experience with it.
How would you expect that effect to vary with those two factors, hunger level and age? Would it be greater or less in a young rat or an old rat? You might say, well, an inexperienced rat, he's more likely to eat anything. But in fact, it's the reverse.
You should know with humans, when you were younger you were probably much more likely to avoid a lot of things. And your parents were always trying to get you to try new things, right? And you say, I don't like it.
My youngest daughter would say, I don't like that stuff, and she's never had it in her life. I knew that because she'd come here from China, so she had never had many of the American foods we were having. But her mother, much older, of course, would try it and like it, and so forth. But as the daughter grows older, she gradually will try more and more things.
But if you make them-- as I pointed out before, if they're hungry enough, neophobia decreases. And again, of course, that's adaptive, because an animal doesn't want to starve to death. So they haven't-- these are innate preferences, though, that we're talking about.
And then Scott discusses another situation of social facilitation, in this case in capuchin monkeys faced with a novel food. They discovered that the monkey was much more likely to eat that novel food if other monkeys were eating near him. And it didn't even have to be the same food, which was the surprising part of the study.
It would be understandable if he saw other animals eating it and it was the same thing. Oh, that's got to be safe. They're eating it, it's not going to hurt me. But they'll do it. They're more likely to eat the novel food, even if the monkeys are eating something more familiar. And I can't really explain that in terms of adaptive behavior.
OK. Let's talk about another social effect on foraging. The ospreys, a bird that forages for fish in the ocean. How can they benefit from hunting, that is, fishing success of neighbors, even though they don't share?
The osprey that brings a fish back to their communal colony doesn't share it with the others, and yet it will affect the others, because-- and there are some data put in the chapter there, Scott's chapter. He presents some data that animals, the frequency that they fly off in the same direction greatly increases if a osprey has brought back certain kinds of fish, but not all kinds of fish.
Why does it depend on the kind of fish? Even if you've not read it, you should be able to think of some reason why it might have evolved that way. Because fish differ in how likely they are to be swimming in schools. If the fish aren't schooling fish-- like the Aioli, for example, in the oceans out here. They tend to be schooling fish.
But flounders are not in schools. So if the osprey comes back with a founder, the other birds will ignore it. They'll just consider that bird a lucky bird, but it's not going to affect them.
But if it was one of these birds-- he doesn't mention herring, but herring are another schooling fish. So if it was a fish like that, then he's much more likely to fly off in that direction because he knows there's got to be a school of them out there, or this bird wouldn't have come back with the fish.
So that gives them some benefit for living in a colony. So it serves as a kind of center of information transfer.
They've looked for the same thing with black-headed gulls, which also nest near each other. And yet they don't respond that way. Even if a black-headed gull is coming back from a pile of food, so there's a lot of it available, the others won't fly off in that direction. So the question is, why are they different?
I have in the margin of this book, which I've had for several years, black-headed gulls are stupid in comparison to ospreys. But in fact, there is a reason why they might have evolved that way, at least earlier in their evolution. They probably fed mainly on food that was scattered. OK, so not likely to be found. So they'd be wasting energy if they constantly flew off in the direction that an animal had come from just because he had food, just because of different feeding preferences, at least for a long period in their evolution. So they've evolved differently from the ospreys.
OK. Group foraging. I want two good reasons why foraging in a group of herbivores may be better for an animal like a bird than foraging alone. And I can give you a hint.
Pay attention to birds right now. If you've ever looked at birds outside, you will notice something happening as the fall goes on. Food is still fairly plentiful, but it becomes less and less as the winter approaches. And when snow's on the ground it becomes much harder to find.
So what change do you see in birds? Birds that you normally never see in groups, in flocks, start flocking. It looks like they're all just flocking because how they're going to fly south together. But in fact, in many cases that's not the reason. You'll see sparrows doing this, you'll see juncos doing this. They all stay around here all winter.
So why would they do that? What are the benefits? First of all, protection from predators. Because when there's a lot more eyes, the predators have finding less food too. So they're going to be hunting. These hawks, the red-tailed hawks around here certainly catch pigeons. They prefer those, because they're bigger and fatter, but they'll catch songbirds too.
So the predator's more likely to be detected if there's a lot more eyes that could see them. And also, if they're in a group, and there's a red-tailed hawk attacking, they're only one a group. So the chances that they'll be the one the hawk selects are reduced. That's a kind of dilution effect that protects animals in a group.
In addition, we mentioned the-- oh, the second one, of course, is they're more likely to detect food sources for the same reason that they're more likely to detect a predator.
It's very interesting watching a large group of birds doing this. All it takes is one seeing the food, and he flies off separate from the others. Almost always the entire group almost immediately is with him and flies down. And of course social facilitation does increase their food consumption, in addition.
This is just an example of group foraging. Here's pelicans foraging in groups. The groups, they tend to be a little smaller. Here's the American White Pelican, one foraging group.
Does the same reasoning apply to carnivores in their hunting behavior? Well, some of the same reasons apply. They have increased likelihood of finding prey. They have increased likelihood of catching them because of cooperative hunting strategies.
And these are examples I can think of. All these animals have been studied, and their hunting behavior has been studied. We know that lions, or at least lionesses, hunt in groups. Wolves certainly do, though the groups vary a lot in size. The wild dogs, the African wild dogs, always hunt in groups. You find some of that in hyenas as well.
And then in the sea mammals, the killer whales, the orcas, hunt that way. And even humpback whales engage in cooperation in their catching of prey. Like they trap herring fish. One of them goes below and emits a string of bubbles, and the bubbles rise up and form a kind of cylinder around the herring up above. And the herring, of course, could swim right through that, but they don't. They avoid bubbles. So they sort of trap them in there. And the humpback whales up above then can feed on the herring.
So it's a cooperative hunting strategy. They obviously have to take turns being the one that creates the bubbles, because they're getting much less food.
This is just examples. There you see a bunch of female lions, the lionesses. And there you see below, a group attack on a buffalo. And they would have very little chance of bringing that buffalo down if just one of them were doing it. It would take at least two, and the more there are, the more likely they are to bring it down.
I put this in just so I can point out that just because they're a group they will not always attack. I mean, what is the likelihood that even three lionesses could bring down that rhinoceros? For one thing, the rhinoceros can run pretty fast. And the other is, the likelihood that he would gore at least one of them, and maybe all of them, are very high. And they can't afford a severe injury. They don't have lioness doctors. So they will simply watch animals like this.
They have to make the decision, is it worth it? What's the cost? If they're super hungry, yes, they will attack even animals like this.
Here's a group of wolves. One will never bring down a buffalo. The buffalo's too big and strong. He can kick very hard with those hooves. But with multiple wolves-- and they often precede this by running, chasing the animal for a long distance. So they tire him out, and that makes him less able to fight them off when they finally attack. And so it does take a wolf pack like this-- this is a fairly large pack-- to bring a buffalo down.
And here's the African wild dogs. You see how relatively small they are compared to that zebra. But again, these dogs are well known for singling out the weaker ones and then chasing them for a very long distance. They have great stamina, and they take turns being the ones closest to the zebra, which takes a little more energy.
So they take turns doing that until finally they exhaust the animal. And then, in spite of their small size, they can kill an animal like this. You can see the zebra there. He will kick out at them if they get too close to him. The dogs are patient. They just run him down until he doesn't have the energy to do this.
And since the year 2000, there's a number of things you can find on the Web using Google Scholar, including a number of theoretical models about group hunting and group foraging.
One article Is about raven scavenging, which favors group foraging by wolves. Why would that be true?
This is the article. And here's what they're talking about. If a wolf pack-- if there's only two, and sometimes three, in the wolf pack, they're very, very likely to be closely related, so they're helping each other. They're helping the genetic fitness of their siblings, usually, or their parents. But the larger wolf packs often include unrelated individuals, and yet they will still join unrelated individuals, will join wolf packs and they'll be accepted.
So why would that be? What would the benefit be? Because in fact, you find that, on average, they're going to be able to hunt better and eat more food if they're not in such a large pack. Because after all, when they bring down prey, the whole pack has to eat.
And they found a reason. Studying these wolves, they found out that if, let's say there's only two, and they bring down food, it takes a long time to eat large prey. And they lose a lot of the meat to scavengers like ravens.
But if they're a larger group, they lose a lot less. There's a lot more wolves to pay attention to the scavengers and drive them off. And they modeled it making assumptions drawn from their studies, and they were able to show that this works. There's a good reason why they would do this, just because of the ravens scavenging. So it just points out factors that you wouldn't think of ordinarily. But in fact the computer models show that it's very likely to be true.
Here was another computer model, but it's relevant to something I'm going to bring up a little later. So if you're interested in the modeling, this is a good one on what we call the ideal free distribution.
How do animals distribute themselves when they're foraging in groups, but groups can go to different feeding areas? Some of those feeding areas are very rich. Some of them are not so rich.
And yet they distribute themselves in an optimal way. It's called the ideal free distribution if you solve it theoretically, where they should be. So we'll be talking about that.
In this one, I mentioned this briefly. This was a model dealing with this kind of problem. When they're foraging in a large group, they don't actually have a clear leader. They do not need a clear leader to be able to function well. Like if they detect food, only one or two birds has to detect the food, and yet it will very rapidly be communicated to all of them because of the types of cues they need to respond to.
Similar things are true of migrating birds. There often is a leader, but the leader is often temporary. It can change. And they keep flying in a concerted way, even without a clear leader. And yet they behave in a coordinated way. If they turn, the whole group turns, and so forth. So this is about that issue. And it's been dealt with also in simulation work.
OK What do we mean by the term optimal foraging? What should a foraging animal be optimizing? Give me a quantitative expression. He should be optimizing the amount of food taken in, the net amount of food, that is, net-- no, that's not fair. Net energy, which is from the food he's eating, but that has costs, too. They're using energy to get it. So the net energy intake per unit time, that's what they should be optimizing, OK?
A foraging animal should optimize energy intake minus costs, and that leads to these kinds of questions. Why should a feeding animal move to another patch of food if he's had a rich patch? Yet we often observe they move to other patches, too. Why do they do that? How long should a dive last, if he's a diving animal, or a diving bird, or a sea mammal? What determines that? Why did they evolve the kind of behavior they've evolved?
I picked these examples from the book to discuss a little bit. The first question is crabs. Why do crabs choose to feed on intermediate-sized mussels instead of the bigger, more meaty, larger mussels? They generally ignore the smallest ones, but they also will ignore the largest ones and pick the intermediate-sized ones. And if they deplete those, then they'll feed on the smaller ones.
Why don't they feed on those big meaty ones? Because they're so hard to open. It takes so much energy. So it's a very simple matter of, remember, energy intake minus costs. So you have to take the costs into account if you're going to explain the foraging of these animals. Here we're talking about crabs.
If we deal with marine iguana, we note that the large ones feed, like this one, they feed subtidally, that is, at high tide they will be out in the deeper water catching food. Here's a whole group of them. Now the smaller ones, in fact, generally don't do that.
In fact, if you keep track of their size, you can put them in three groups. The big ones like this, the intermediate ones, and the small ones. The small ones all feed at low tide in the shallow water. None of them feed subtidally. And only in the intermediate size you'll see some of them feeding both situations.
So what is the reason? Very simple. They lose body heat. Remember, these are reptiles, and they lose body heat. And it's colder there in the deeper water at high tide. So that is the cost, because they have to make up for it. They have to eat rapidly enough that they can, by afternoon, when the sun is out, they can sit on the rocks and heat their bodies up again.
And if they're smaller and they have to-- and they're not catching the food as well, especially because they're slowed down because they're getting cold, they might not warm up in time. So it's much more efficient for them to feed in the less productive shallow water.
Let's talk about, now, the foraging birds again, in this case dealing with the redshank. I'm asking here, why do they visit less productive patches in addition to their focus on the most productive areas for finding the large worms that they feed on in the ocean bottom?
And the reason they do it, apparently, is to get information about food availability. They find a need to know what's the relative density of food in all the nearby feeding areas. And also they need to know how many birds are there at each one. Because no matter how rich it is, if there's already a huge number of birds there, the amount one animal is going to get is going to be reduced.
These are redshanks. There's one showing him by himself. You see the kind of shallow water that they look for those worms in. Here he's poking below the water to pull out a worm. Here's a group of them, and they normally forage in groups like this. So we talk about the ideal free distribution in a description of foraging by groups of animals.
So what do we mean by that? An animal will tend to go to a less rich food patch if joining a group of animals at a richer patch would reduce the amount of food per animal there to a point below what it could get at the most rich patch.
You say, well, how could he possibly know that? They do. And they do it by this sampling method that I'm talking about. They're collecting information. None of them will stay at that rich patch all the time. They're always flying away a certain percentage of their time, sampling the other areas. So they know what the average is for that area.
So they've evolved. So they distribute themselves in an optimal way to avoid excessive competition. And this guy Charnov developed the ideal way they could do it if they had all the information. And to make it more practical he modified it.
So they know now that an animal seems to sample food sources in a region enough to know the average density of the available food items, and it changes its foraging location when food density drops down to or below the average density of the area.
But there is an additional factor that needs to be brought up. And that is that dominant animals often will exclude the subordinates in the rich patches. So the animals that are more likely to be a less rich patch of food early on, even though there's still a lot of worms left at the rich patch, are the less dominant animals.
They're each optimizing their feeding success, because they have to take into account how much time they're going to be allowed to forage by those dominant animals. OK
And this is from a figure Scott publishes, where there's two patches of food. The profitability is on the ordinate, so you see one patch is a lot more profitable than others. But how profitable it is depends on the numbers. You can see if there's is between one and five animals, you can see if there's five, it's becoming less profitable. It drops down to the point that is labeled x there. And it's just as profitable for him to be in the other, less rich patch at the point labeled y there. And that, of course, depends on numbers of animals there, too. And that would affect the shape of these curves.
That basically is why animals will move. They collect enough information to be able to judge profitability of each of those areas. And they also seem quite aware of the number of animals at each place feeding. They know the rate that the food is being depleted.
So what do they do if there's a predator near? Just because there are predators around-- there's often predators around, you can't stop eating. So when you study that, study the conflict they have, it's been studied quantitatively in these two types of gobies, the sand gobies and black gobies. It's observed that feeding decreases, as you would expect, if a predator is detected nearby, because they have to spend more of their time avoiding that predator or hiding from him.
But that effect is less if the animals are hungrier. In other words, they will be more risky in their behavior if they're very hungry. But it's also less if they're better camouflaged. And the sand gobies are better camouflaged than the black gobies, so they were less affected by the presence of a predator. They have an innate difference. It's as if they knew that they're camouflaged and they're going to be harder for the predator to see.
So I'm asking the question here, is this ideal free distribution relevant to population control at all? The answer appears to be no, because the models, anyway, of it don't attempt to extend overall population control, but only the small foraging groups. It would be too much of an extrapolation to extend it to an entire population.
That has been studied scientifically, and we know that overcrowding has been studied extensively in rats, but there are some studies in other species as well. We know that fertility goes down, they end up with impaired immune systems, so you get more illness, more infections. They become more irritable, they fight more. If you look at their adrenal glands, you see that they're secreting a lot more adrenaline. They have larger adrenals.
And you also see in these groups really sudden population declines, not just because they're being caught by predators, but because of these other factors. And this has been observed for many small mammals that tend to reproduce very, very rapidly. And they tend to move towards an area that's maximal for what the environment at that time can support.
But if they become overpopulated, you get population crashes. It's very well known for some small mammals. You probably know about the lemmings, that when they become overcrowded in Scandinavia, they migrate in large numbers. And sometimes they seem to get confused in their navigation, ands they will swim out to sea when normally they're just swimming across the fjords to get to another area where there might be fewer lemmings.
But sometimes they just swim out to sea as if they're committing suicide. But it appears to be a navigational problem. And it could be because of malfunctioning nervous systems.
OK. I don't want to discuss murder right now. We can do that later when we talk about sociobiology. But what we'll do is, I want to get started talking a little bit about anti-predator behavior, which is the next topic, because I have a couple of nice videos I want to show, especially one about animals escaping from predators, so you can see that, the number of predator-prey relationships.
Scott starts out talking about anti-predator behavior in his Chapter 7 by distinguishing between primary and secondary defense strategies of prey animals. And these are defined like this.
Primary strategy simply decreases the probability of being attacked. So if he's camouflaged or if he has good hiding strategies, then the probability he'll be attacked is reduced. He times his foraging. A hamster comes out only when the shadows are long in the twilight period. So he doesn't come out when he's easier to see. And he comes up when there's still some light, but the shadows are long. It's easier for him to hide. Animals are active at night or day for similar reasons.
Then you have group foraging, again, that decreases the probability that any one animal will be attacked. And then of course you have warning calls. Sometimes they're responding to warning calls of another species.
Secondary strategies are when an attack does occur, they need ways to get away from it. Fleeing as rapidly as possible, and dodging the predator chasing them, is extremely important. And you will see in the video I'll show next time how successful that can be. It tires out the predator, greatly increases the chance of getting away. Having very high endurance is important. They have to be able to outrun a predator and keep running without tiring, so the more endurance they have the better.
Some of them will just have a play dead strategy. And the opossum's well known for that, but there are other animals that do that also. Why would they do that? Well, because if they act like they're dead and they're totally still, it doesn't evoke predation behavior as much. The predator is likely to lose interest. Or he might just say, well, I can come back to this one. It's dead anyway. So we will see some of that.
OK. What is countershading? And I'm asking another question there besides what it is. Why would cephalopods need a countershading reflex?
Well, here's an example of countershading. There's actually two birds here. How many do you see? You see this one because he's got countershading that's opposite to what it would be normally. It's a model.
Normally birds are lighter on the underside, darker on the upper side, because when you're above a bird-- let's say you're an eagle, you're hunting for small birds flying down below-- if those birds are dark on top you're less likely to see them because the ground is generally a lot darker than the sky above. But if the bird of prey is below and he looks up, he's less likely to notice birds if they're light on the underside.
So with reverse countershading, it makes this bird stand out. But the other one that's in there is camouflage. And so it's very hard to make out exactly where it is, at least in the black-white photos.
So these are a year or two ago. Jenny Wu was in the class, and she pulled these off the web. By the time I got to the topic, she was already doing the reading, and she found these very nice illustrations.
The animals in these pictures don't move. They're very hard to see. And I point out what's there.
It's not that easy to tell that that's the body of a spider right there.
Here the crab is sort of easy to see, but if you're just glancing around and there's a lot of unevenness in the gravel bottom of the water, it's very hard to see that crab.
And here again, if you're glancing around, you don't notice that frog there that easily.
And here, the dragonfly, it's only because I tell you there's a dragonfly there that you will probably make out, oh yeah, those are some legs there, and there's a head there.
And here's some other examples. There's a bird in this picture, a leaf warbler, well-camouflaged, he looks just like the leaves.
Here the jaguar, a predator, is pretty well-camouflaged. Hard for prey animals to see him when he's still. That's why a predator will spend quite a bit of time still. And when he does move, he moves rapidly to a new place, waits again.
And here there's a caterpillar on that leaf. Almost impossible to see, but that's the outline of the caterpillar there. If you don't know he's there I don't think you'd notice it at all in the picture.
Just like here the grasshopper, taken in Colorado, very hard to see. If you look very carefully, you'll see that there's an eye right there, I think. That's camouflage.
So we'll start the class next time about predators who-- because predators develop search images. They have an image of the prey they're looking for. And octopus and squid have developed an incredible ability to counter that ability by changing their appearance. And so we'll start with this next time.