Flash and JavaScript are required for this feature.
Download the video from iTunes U or the Internet Archive.
Description: This lecture covers how neurons respond to motion and the effects of lesions on motion perception. Other topics also discussed are the ability to determine structure from motion, apparent motion, meta contrast and brightness masking, optokinetic nystagmus, and the accessory optic system.
Instructor: Peter H Schiller
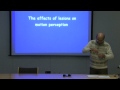
Lec 12: Motion perception a...
The following content is provided under a Creative Commons license. Your support will help MIT OpenCourseWare continue to offer high quality educational resources for free. To make a donation or to view additional materials from hundreds of MIT courses, visit MIT OpenCourseWare at ocw.mit.edu.
PROFESSOR: All right. Good afternoon, everyone, again. Today, our session is going to be on motion perception and pursuit eye movements. Now, I want to remind you that to our next class next Monday is going to be a whirlwind review. And then the next Wednesday we are going to have the midterm exam on what we have covered so far in the course. And as I've mentioned already to you a couple of times, that is going to consist of a series of multiple choice questions in which you have to circle the appropriate choice for each question.
Then the following week, which is going to be November 28, is when Chris--
AUDIENCE: October.
PROFESSOR: Huh?
AUDIENCE: October 28.
PROFESSOR: Sorry, October what?
AUDIENCE: 28th.
PROFESSOR: October 28. Yeah, October 28-- that's when Chris Brown is going to start the second half of the course on audition. So that is the general plan at this point. And again, I'm going to send around an attendance sheet. So just put your name on it and send it around, please, for me. Thank you.
All right, so I think you're going to find talking about motion perception a lot of fun. I have several demonstrations. And it is certainly have a very interesting topic. And I'm going to highlight that by first of all telling you what we are going to cover. And then we are going to talk about the basic mechanisms of direction selectivity in the visual cortex.
So first of all, as I've said, we're going to talk about the neurons in the brain that code motion. I'm going to talk about it mostly for in the cortex. But I would also say a few words about direction selective cells that you find in the retina that we had mentioned before.
All right, so we are going to then discuss briefly the possible mechanisms that make it feasible to process motion. And then we are going to look at what happens when you make various brain lesions in determining how well you can process depth. Then we're going to talk about a very interesting topic, which is structure for motion. And then further pursuing that, we're going to talk about apparent motion.
And then we are going to talk about an interesting temporal effect which is called metacontrast. And then we are going to talk about optokynetic nystagmus, where we link the visual input to the generation of pursuit eye movements. Then that is accomplished by the so-called accessory optic system that I will describe to you. And that is also the topic that, if you remember, you will be writing a paper on. Lastly then, we are going to have a summary.
OK, so let's look first at the neural responses to motion in the cortex. Now, one of the most remarkable features of the visual cortex and extra cortical areas, as I had already mentioned, is that the majority of cells in these areas are direction selective, meaning that they respond to one particular direction of motion, but not to the opposite direction. Now, this is extremely evident in the visual cortex, where the so-called simple cells, virtually all of them are direction selective.
So that's one of the major transforms, if you remember, of the input that you have from the lateral geniculate nucleus that goes to the cortex. That input is just from single cells, on and off single cells, midget and parasol cells that have circular receptive fields that centers around antagonism. And when they get up to the cortex, a transformation takes place by virtue of intracortical circuits that turns these cells into direction specific ones and cells that are orientation specific.
So let's look at this first by starting with a method so that you'll understand exactly how this is done. In this case, what we are going to do is we are going to have a photo cell. Let's just look at the top one here, A. You're going to have a photo cell that responds both to a light edge and a dark edge. And so when this bar moves across this photo cell, it will activate it. And what they're going to do-- we are going to move it across in one direction. And then we are going to move it back across in the other direction.
And this is shown here. This is a time scale. And it shows that when this edge goes across, it activates the photo cell, gives it the beep. And then when the trailing edge goes across, it also does the same thing. So this is the light edge response. This is the dark edge response. Now, when you're going back the other way, you're going to have, again, the same arrangement. In the opposite direction, the light edge responds first. And the dark edge responds second.
Now we are going to make a change so that it mimics the organization of simple cells in the visual cortex. We're going to put two photo cells, one which responds to the light edge and a dark one which responds to the dark edge. So now when this bar goes across and activates these two photo cells, what happens is, because they are not all centered exactly in the middle, it takes a longer time to activate the two successive [INAUDIBLE] when the bar goes across this way and a short time when it goes across the other way.
Now, the opposite happens when you have a dark bar, because then the dark bar doesn't activate the light sensitive photo cell. It activities the dark one. So it takes a little longer to start that. So that then shows you the temporal arrangements of these responses if when the photo cells are arranged in this fashion. Everybody understand that?
OK, so now we are going to do this for the real. We are going to look at a cortical cell. And there are several different subclasses of simple cells. And the first subclass we can refer to as an S1, S for simple, OK? So the simple cell there is an example, very similar arrangement.
Here's the receptive field of the cell, undefined. And you either put a light edge across back and forth or a dark edge back and forth. This is the light edge response. This is the dark edge response. Now, you can readily see what happens here, which is quite remarkable. The cell responds only when the bar moves upward across the receptive field and does not respond when it moves downward. So this cell is over 100% direction selective. Got it? All right.
Now, the same thing happens to the dark edge, all right? And so this cell is one that responds only to the dark edge, OK? In this case, it's the trailing edge. In this case, it's the leading edge. Now, at the bottom here, you draw out what the receptive field looks like. This is in 10th of degrees. And so this is pretty much just the spatial response of the cell. And it only responds in this direction. There's nothing drawn in the other way.
Now I'm going to show you another cell, which is going to be a so-called S2 cell. Now, this cell is different in that it responds both to the light and the dark edges. And the fact that they're-- in this case, a light bar, in this case, a dark bar, the fact that they're-- displaced temporally mean that they're side by side for the light and dark responses rather than overlapping. And that, of course, is the definition of a simple cell. And so if you draw out the receptive field of this cell, once again, the cell is almost 100% direction selective. And this is what its response looks like in space.
Now we are going to look at yet another one. And this is going to be a surprise. It was certainly a surprise when this was discovered, because it's still not understood why we have these kinds of cells. Here is one-- you can look at that-- that responds to a light edge in this direction and a dark edge in this direction, OK? So both subfields are direction selective. But they're direction selective in opposite directions, as depicted here.
Now why on earth would you have cells in the visual cortex that respond selectively to light and dark edges in opposite directions? That's something you can contemplate. There is no definitive answer to that, and so I'm not going to belabor it any further?
Now what I can do is I can provide you with a summary. I showed you this one, this one, and this one. Now, you don't have to worry about those. But I would like you to look at this last one, which is a complex cell. In a complex cell, the light and dark edge responses are in the same location, space-wise, as shown here. And this particular complex cell that's a real cell is 100% direction selective.
Now, it's true not for all complex cells, but the majority of them that they too are direction selective. So I can safely tell you that one of the major transforms that happens in the brain in vision is to convert the signals form the retina into direction selective cells. And therefore, we can only surmise that to have direction selective cells is extremely important for us to be able to process motion information in the world.
So now we are going to move on and look at extrastriate cortex. And we already talked briefly about MT and MST. In these two areas, we have even an stronger disposition for cells to be direction selective. And here's an example of a cell in MT-- in this direction of motion, a vigorous response. This is a cumulative response. In the opposite direction, it actually has some inhibition, as you can see here and here.
Then if you recall from MST, which is an area yet further removed from area V1-- it's the middle superior temporal area-- what you find is, again, very strongly direction selective cells. Most of them-- maybe not all of them, but the overwhelming majority of them-- are direction selective. But they have much, much larger receptive fields than cells do in MT.
And as I've mentioned to you before, one of the basic rules is that as you progress from the V1 to higher cortical areas, the receptive fields in these progressively higher areas become bigger and bigger and cover more and more of the visual space. So here you can see this is shown in terms of the visual field here. This is way out. This is 20 degrees just from here to here. The receptive is gigantic, actually. And there's a beautiful, very lawful arrangement and even though many, many cells feed into this particular cell from earlier portions of the visual cortex, they maintain or get a specific input from all of the cells that have this direction selectivity.
Now, whether this direction selectivity is a product of the input from the cells, which have the same kind of directions, like many, many of these cells-- maybe 20 or 30 or 40 or 50 of them, OK?-- which have the same orientation and direction, or whether orientation and direction selectivity are created anew in MST is something that can be debated. It is still not 100% certain. So the only thing we have to worry about this time is to realize-- maybe not even worry. That's too strong a term-- is to be aware that there is prevalence of direction selectivity in both MT and MST. It's very beautiful and very, very specific, as you can see it here, even when you have a huge receptive field.
So that is the basic story then. And now we can ask the question, how on earth is direction selectivity created? Now, there are several models that have been proposed. And most of these models-- I'll show you a simple one just so that you have a sense for it-- most of these models assume, I believe correctly, that you need to have inhibitory circuits that are selective for direction to produce this specific response in these cells.
Now, the amazing thing about all this is that already in the retina you have some cells that are direction selective. And these cells I'll talk about a bit more when we talk about the accessory optic system. These cells in the retina are not very numerous.
In the rabbit that has approximately 350,000 cells in each retina-- can you imagine a little rabbit? And it has 350,000 cells. We have a little over a million, OK? And among those 350,000 cells, about 7,000 are the so-called cells of Dogiel. And actually, I'll come back to that. And those are direction selective cells.
So a very clever experimentalist examined these direction selective cells in the retina, a fellow called Nigel Daw. And he asked the question, what can I do to determine what the mechanism is of direction selectivity? So he did an experiment that sounds simple. But let me tell you, it's a very difficult experiment.
When you put your electrode into the retina, you can't see direction selective cells. So you're on a hunting expedition. So you keep recording from cell after cell after cell. And if indeed only 7,000 among the 350,000 are direction selective, it will take you quite a while before you manage to record from a direction selective cell. So it's a lot of very hard work.
So anyway, if you do that when your hypothesis is that some sort of inhibitory mechanism is involved, then you can do an experiment and say, well if it's inhibitory mechanisms, probably some sort of GABAergic system, we need to put some agent into the retina that blocks inhibition. We talked about that before, that that can happen. And so in this case, what they did-- let me come to that first.
Let me see. Here we are. I'll come back to the others in just a minute. What we have here is one of these cells. And we move in this direction here and in this direction for this. And you can see that this cell is very strongly direction selective, maybe 100%, but maybe 80% or 90%.
Then he injected picrotoxin into the eye that blocks GABA. And lo and behold, direction selectivity is virtually eliminated. And then when picrotoxin got washed out, there was recovery, all right? So now, on the basis of this finding-- it's a beautiful finding-- it was established that indeed, inhibitory circuits play a central role in giving rise to direction selectivity.
And you can imagine that in the course of evolution, the pressures had to be tremendous to create these direction selective cells, because the circuitry is very complicated. You have to create these inhibitory neurons which inhibit in a particular direction rather than just randomly. So that's what was done. And so I'll show you one model that describes this.
You have a bunch of-- this is for the retina. You have a bunch of receptors here. And they activate a retinal ganglion cell here. But they also connect with an inhibitory interneuron in one direction, in that direction. So now if you move a bar in this direction, as shown here, you get no response. But if you move a bar across the other way, you get a vigorous response, as shown by the action potentials here.
So this is a fairly simple model of creating direction selectivity by virtue of inhibitory interneurons. So that then was also examined in the cortex. it was found that there too inhibitory interneurons play a central role when direction specificity is created in a cortical cell. So that now makes me come back to a further analysis of the basic nature of motion. Can we logically divide motion into several types, or what?
And so it was proposed by a wonderful scientist called Wurtz at the National Institutes of Health that he could-- he decided that you can distinguish between planar motion, circular motion, and radial motion. Planar motion is when something moves straight. That's what we've talked about so far only. And of course, you can do that. In this case, he shows four different directions.
But of course, there'd be all kinds of directions that are not vertical or horizontal but are diagonal. And there are many, many cortical cells. Some of those actually I showed you had orientation cell activity that was at an angle. So you have a huge number of different angles. And these cells hypothetically then would respond to planar motion.
Then you have another type of motion, which is called circular. And let me tell you, when you see circular motion, obviously, if you were to be spun around or something, you'd see it. But it's not uncommon to see something rotate. So if you have a spinning wheel or if you watch a tire rotating in motion, that's circular motion. And so the hypothesis is that there should be cells in the brain that selectively respond to circular motion, either clockwise or counterclockwise.
And lastly, of course, the so-called radial motion-- and you encounter that just about every day. Whenever you drive, you see trees. Or if you're driving out in the country, you see trees going past you, so to speak, as you drive forward. And that would be motion which would be outward. Or if you were to back up like this, then you would get this kind of motion where things would come together, OK? So that kind of motion is certainly one that you need to somehow code.
And so another question came up once he came up with this hypothesis that these different kinds of motion-- the question is, are there cells in various parts of the brain, especially in higher areas like MST, that are selective for these three basic types of motion? And so they began to do an experiment. And I'll show you some summary data here.
Here is a cell when you study planar motion. And these are the eight directions of motion that were studied-- sorry, four directions of motion on the top-- then the circular one on the next two, and the in and out motion at the bottom. This particular cell seems to be specific for planar motion from right to left. Now here is a cell that is selective to circular motion, OK? And lastly, here is a cell that's elective to radial motion.
So if you only had recorded in your life from three cells in MST, and it happened to be these three cells, you would say, ah, my hypothesis is right. There are three kinds of cells in MST that respond selectively to these three classes of motion. So everything is incredibly beautiful and logical.
But of course, that's not how you do your experiment, is it? The way you do your experiment is you record from MST in this case, and you record from many, many, many cells. And you test them just like this, OK? And if you do that, you realize that this is just an example of three cells that were specific.
It was found that 40% of the cells responded to all three kinds of motion. 305 responded to two types of motion. And only 20% of the cells responded to one type of motion. So therefore, these cells, as is so often the case in the visual cortex, are not unique and specific to analysis of one thing.
These cells can tell you about all three of those. And what happens in reality then is that when something moves, you activate thousands of cells in MT, MST, and visual cortex, maybe tens of thousands. And it's some sort of complex relative activity that then eventually gives rise to the fact that you say, oh, yeah I see planar motion. Oh, yeah, I see a circular motion, or I see in and outward motion. So it's not a specific single cell that tells you or gives you that impression, but it's the concerted activity of thousands of cells even for the simplest of cases.
So that's the way it works. It's a complex business. And the idea that was popular at one time, namely that you have feature detectors in the brain, has become downgraded progressively with increasing research.
As I mentioned to you before, when we talked about to extrastriate cortex, the initial idea was when the various extrastriate areas had been discovered, that each area specializes in one particular thing. And then it turned out that that is not the case. There may be shifts or trends, but in each area, many different things are analyzed and thousands and thousands of cells are active whose relative activity is somehow what gives rise to the percept that you have.
All right, so that then is the essence of the layout of the responses and the models that this model that [INAUDIBLE] told you about, or something similar to this. It may be a bit more complicated. And some people have hypothesized that there are temporal delays necessary to produce this thing. Yes?
AUDIENCE: Sorry. There was a 10% missing in the breakdown.
PROFESSOR: Sorry. Are you talking about this?
AUDIENCE: Yeah.
PROFESSOR: Well, that's-- 10% of the cells were unclassified or we're not direction selective. These they could with certainty say what the situation was. And maybe some of those cells they lost while that we're recording from or they didn't have any particular direction selectivity. And because of that, they couldn't come up with 100%, of course, yeah? Yes, you had a question.
AUDIENCE: It was the same question.
PROFESSOR: What?
AUDIENCE: It was the same question.
PROFESSOR: Same question, OK. Very observant-- good for you. All right, so now we are going to move forward and we're going to look at the effects of lesions on motion perception. One of the ready ways you can assess to what extent various higher cortical areas play a role in various neural mechanisms is to either reversibly inactivate them or to remove them by making lesions and then determining what happens.
And if you remember, I talked about this a bit. I told you, for example, that the hypothesis that was made initially about area V4 is that it's a color area. That was a single function hypothesis. And then it turned out that when area V4 was removed, it was found that there was only a moderate to mild deficit in color vision. But there were all kinds of other deficits that we had discussed in previous sessions.
So now we come to the question about, what about motion perception? When we talk about emotion perception, we of course are dealing with temporal things, because motion involves successive activation of cells. And because of that, it's also very important to study how well we can process rapid successive presentations as, for example, in flicker.
And that brings me, of course, to the story-- and we will talk about that in a minute-- that in the olden days, when movies were first invented, the rate at which successive frames were presented was 16 Hertz, which was fairly close to having a sensational of flicker, all right? And because of that, what happened when a guy asked a girl to go the movies? How did the guy ask the person to go to the movies? Can anybody remember that? That's an olden thing. The guy would say, hey, how would you like to go to the flicks tonight? OK, you've heard that expression, go to the flicks, right?
Well, nowadays we are not aware of flicks anymore. And there are two reasons for that. Anybody know what the two reasons are why no one can see clicks in the movies and you no longer go to the flicks anymore but you go to the movies instead? Yeah? What are the two reasons? Anybody know?
OK, well, let me tell you. The first is that the rate of the number of frames per second had been increased to about 24, OK? But that's still slightly below the effusion. OK? You can still see some flicker. So how do you get rid of it?
Well, let me tell you, some incredibly clever guy-- some things are really, really clever. I'll tell you what this person came up with. He said, all right, what we'll do is we're going to create a shutter which is round. And it's going to have one opening here. And then we're going to have a second opening here. And then we're going to have a third opening here.
So this goes around. And each frame you're going to show three times. And then as this goes around, you go to the next frame, and you show it three times. So actually, if seeing frames 24 hertz, you see it 3 times 24, OK? But each frame is shown three times.
Now, to be even more clever, the way they did this, they took two of these circular shutters. And one of them moved in this direction and the other in that direction. They sort of equalized from the center out as the light was expose from the projector. So that then reduced the rate of flicker.
So therefore, we can next ask the question, what is the flicker rate at which you no longer can see anything? I mean, do you want to see flicker. Or do you not want to see flicker, and so on? And so experiments were done, hundreds of experiments in which a psychologist studied flicker rate, varying contrast, varying color, doing this, doing that, and then varying the spatial frequency and generating curves to see what they look like.
So that's the process. And so what we can do next is to examine this. And one way to do this, for example, just to give you a sense, you can have, for example, a bunch of random dots, just like we had for the [INAUDIBLE] stereogram. And this would be done with a monkey, for example. The monkey fixates. And then you start something in motion-- ready?-- like that. And the monkey sees this and has to make a saccade to it. And when he does so, he gets a drop of apple juice for reward.
And then what you can very are two things. You can vary the frequency. I'm showing another one, much higher frequency. Bang-- the monkey goes there and gets rewarded. Or you can-- and/or, actually-- you can vary the contrast of the display, OK? So once you have a sense of when you get fairly close to sort of the edge of things, then you can also vary the contrast.
So now let's ask the question, what happens when you remove area MT and when you remove V4, OK? So think about that for a second. And then I will show you the actual data, all right? OK, here we go.
Here is an example. This is motion detection. And here we vary percent luminance contrast at a fixed frequency. You get the same effect the other way. I'll just show you this one. And you can see very readily that the V4 lesion caused no deficit at all, but the MT lesion produced a huge deficit, OK? So clearly this establishes the fact that area MT plays a central role in the processing of flicker information in being able to analyze rapid temporal events.
And now we can ask the next question. What happens when you actually do just stationary flicker, all right? So when you do stationary flicker, you can do that in two ways. You can do an on off flicker, or you can do a flicker between two colors, for example, all right? And you can see why that would be interesting. Because if you do flicker between two colors, like red and green, if area V4 were important for color processing, then a lesion in that area should mess up that kind of flicker. So that's the way to do it.
So let me show you first of all how you do this experiment. Here we have it. You have a bunch of LED's. Why does one have LED's instead of just doing this on a monitor? Any thoughts? Yes.
AUDIENCE: The frequency at which those [INAUDIBLE].
PROFESSOR: Very good. The reason you do it is because your typical frequency rate is the alternating current rate, meaning 60 Hertz. Now, nowadays you can of course buy devices that can double it or even quadruple it. But back then it was easier to do it this way. And it still is, because you can vary these flicker rates in small amounts.
Now, each of these LED's, actually, for this particular set of experiments, is one that has both red and green in it, OK? So you can flicker between on and off, let's say for green. Or you can flicker between red and green, all right?
So that's what we are going to find out. What happens when you do these kinds of flickers, red green as well as on and off? And ask the question, what happens when you make, again a V4 lesion or an MT lesion, OK? Now think about it for a minute, as to what you would expect in terms of the differences between on and off and red green flicker and possible differences in the lesions that cause a deficit in this, OK?
All right, ready? Here we go. There is the flicker. You can see that. Now, the way that really works is that the overall flicker-- I'll show it to you again-- is done in such a fashion that the mean illumination level is the same so that you cannot tell the location of the flickering LED on the basis of a lumen exchange. You can only tell on the basis of the fact that it flickers or it doesn't flicker, OK?
All right, so we are now ready for the experiment. And here it is. On the right, I'm showing you a green on and off flicker. And you can see that there's no deficit at the V4 lesion, but a gigantic, absolutely gigantic deficit at the MT lesion site. And then very surprisingly-- to some people at least who were convinced that V4 is a color area-- when you do that for a red green flicker, first of all, notice that the flicker rate that you can detect is much lower-- OK, this is the ratio of the flicker, much lower, OK?-- than it is for the on off flicker.
And secondly, again, there's no significant deficit with the V4 lesion. But there's again a major deficit with MT lesion. So area MT is essential for being able to process both red and green flicker as well as on and off flicker, all right?
So bear that in mind as we are going to now progress to the next stage of the experiment-- set of experiments I should say. And the next step I'm going to talk about is structure from motion. And that one is a very important attribute that we have. We can reconstruct structure by see nothing but motion. And I'm going to show you an example of that.
OK, let me tell you, this here actually is a scene, a made-up scene, that's outside. And somewhere in here we have ladybug hiding. But the ladybug is not in motion. And you know, ladybugs have these spots on their back. And there's a ladybug here. And I'm going to tell you that there's a monkey watching this, hoping that he could catch the ladybug to have it, because he loves to eat ladybugs.
Are you ready to catch her? OK, here we go. Ready? The ladybug after a while got tired of just sitting there and began to move. And that's a dead giveaway, because you have all these cells in your brain that selectivity see motion and flicker. Are you ready? Here we go. There's the ladybug. See her?
OK, so the monkey is happily eating away the ladybug, all right? So here is a good example of how incredibly useful motion information is that you have in these visual cortical areas. Now, I would bet that if this monkey had had his MT removed, he wouldn't have been able to catch that ladybug.
Now, another set of experiments carried out, beautiful experiments, were carried out by a fellow called Johanson-- I think that was in Sweden-- who did a very clever set of experiments. He wanted to analyze how well we can process motion information and derive structure from it. And what he did was that he put little teeny little light bulbs on about 13 points in the human's body.
He put some here on the elbows, put some at the hand, put some at the head, and so on down, 13 of them. And then he turned off all the lights, so the only light was that little spot of light that was on these 13 spots of light that were on the person. And then he took a movie to see what happened when this person started to walk.
And he discovered something amazing, that we are incredibly capable of reconstructing shape from minimal motion information. So are you ready? OK, here we go. So there it is. There are your 13 little spots of light. And now I'm going to set it in motion. Ready?
So what do you see? You see a person walking on this very minimal information. And you can even tell-- who would say it's a male and who would say it's a female? Those of you who say it's a male, raise your hands. OK, it's virtually everybody. Right, very good.
Now, so what he did which I don't have a movie of, which is quite remarkable, is that he took a couple, a male and a female and had them arranged in exactly the same way-- each had 13 of those bulbs-- and had them dance. And when you looked at that, you had no trouble telling which was the male and which was the female. Amazing. So we are able to reconstruct a tremendous amount of form detail on the basis of extremely limited information about motion.
All right, so that then ends that session. And we're going to go next to what is called apparent motion, all right? So you're going to have various forms of apparent motion I'm going to tell you about. And the first one is that if you show these two dots, if I flick them back and forth, you have a very strong sense that the thing is going back and forth, right? You don't see one, two, going on and one going off, because of the right temporal succession. But you see them as just jumping back and forth.
So now what we can do-- we can enlarge on that and carry out a series of experiments on the bases which can make some inferences as to whether areas V4 or MT are contributing to this. So this is going be fun for you. And I want you to pay close attention.
Now, in this kind of experiment when it was done, first, this stayed on all the time. And these two dots came on first. And then these two dots came on second. Now, if you do that, you can see either of two types of motion. You can either see what we call zigzag or seesaw. Seesaw is going to look like that, obviously, and zigzag like this, OK? So that's what I'm going to show you.
And once I set this in motion, you will see it one way or the other. Then if you see it's, say, seesawing, you can take your finger and move it horizontally back and forth on top, say. And then in short order you're going to be seeing this. You can reverse the sense of motion, all right? So are you ready for that? OK, so here we go.
If you see it one way or the other, try to move your finger back and forth to change it to see it. If you see it horizontally moving, move it vertically. If you see it vertically, move it horizontally. And then you can see it shift. Everybody able to reverse it? Well, keep doing it. And you should have no trouble reversing it if you move your finger back and forth. This is a bistable situation. And people can readily see the switch. OK, most of you seeing it switching? All right.
So now we can ask a whole bunch of questions. And the way to put this question in general is we can ask, is first of all, is this something that happens locally, or does it happen everywhere when you're looking? So how can you do that?
So let me show you. What they we do here instead is we can put up a bunch of them, OK? And they're quite far apart from each other. And depending on how close you are, they may be as far as a centimeter apart in the visual cortex for the activation.
So now what we're going to do is we're going to move them back and forth just like I did before. And the question that you want to ask yourself-- am I going to see these all move the same way vertically, say, or see all of them move horizontally, OK? So here we go. Are you ready?
Basically, the rule is that they all move the same way. And then they shift. Again, you can use your thumb or your forefinger. And if you manage to make it switch, they all will switch at the same time. OK, everybody manage that?
All right, so now the rule is that even though the activation is very far apart in V1 from here to here, there's some organizing principle that makes you see everything a particular given way. And they shift. They all shift. Now, that's a very interesting arrangement. And you can wonder, what is there that can tell the visual cortex how to see it and insist that you got to see it the same way?
Now we can move on. And you can ask the question-- so far, what I've shown you is they're all the same-- sorry. That's my fault. They are all the same size. And they are geometrically arranged, OK?
So now what happens if instead, we do it differently and we make them different sizes and random locations? What happens under these conditions? Now think about it for a minute. And then I'm going to show it to you.
And once again, even though they're different sizes, different locations, they all go the same way. And when they shift-- again, use your forefinger to try to make it shift. And when they shift, they all shift at the same time, OK?
All right, so now we can move on and ask some interesting additional questions. We can ask the question, how important is color information for you to see it go one way or the other? All right? OK, so to do that, here we have the top two are colored red and the bottom two are colored green.
Now we're going to move these back and forth. And the question is, will you see them move horizontally because of the color information or what? So the question is, again, are you going to see zigzag or seesaw? OK, are you ready?
And you can readily see if, again, you can readily shift it again with your forefinger, that color doesn't seem to be an important determinant of the direction in which the apparent motion will take place. Everybody follow that? Well, now think about that. How can we do this experiment to be more convinced that color for some reason doesn't seem to be important in how we see apparent motion?
Well, let me tell you how you do it. Here is an example. Here we have again four rows and four columns, all right? And the first, one A and C, the colors are horizontal. And in the second one, B and D, they are vertical. So if color plays a role, we should be able to see these, the first and the third rows go in one direction and the second and the fourth row go in the other direction. So let's examine that. Ready?
And you can see that they're all going the same direction anyway. When they switch, they switch in the same direction, meaning that indeed you pay no attention to the color itself, OK? Some mechanism is involved in which the apparent motion you see does not play a significant role in color.
Now we can do another experiment and see what happens when instead of colors, we use small shapes, X's and O's which are horizontal in the first and the third row and vertical in the second and the fourth row. Will this succeed in breaking up the unity of apparent motion? So here we go. Ready?
And you still see them all the same way, meaning that you don't pay attention to the small differences in shape. OK, so now what I have shown you so far, remember, has something to do with the midget and the parasol systems for the test. The midget system can process fine detail. And the midget system can process color. The parasol system does not process fine detail and does not separately process color. So we'll come to that in a minute.
Now let's ask the next question. What happens if you vary the size? OK, again, the first and third row, the size difference is horizontal. And the second and fourth row, it's vertical. OK, so let's see what happens.
Here it's a bit unclear, OK? So as you see, some of them go one way. Some go the other way. And some of you may see it all go the same way, depending on how far you are. So let's change the ratio so it's much, much bigger. And if you do it now, what you see is dramatic. You see horizontal motion in the first and third rows and vertical motion in the second and fourth rows. Everybody see that?
OK, so we can enhance on this maybe a little bit by adding these together, adding size and color and shape, quite large differences in size and shape and color. Then if you do this, you will indeed have fully succeeded in breaking up the unity of motion, with the first and third rows going horizontally and the second and fourth rows going vertically.
Now then there's one other important factor that we need to consider about this. And that has to do with proximity. So in this case, what we're doing now-- in the first and the third rows, we have the horizontal dots closer to each other. he second and fourth rows, we have the vertical dots closer to each other. So if you do that, you're going to ask the question, how important is proximity? And if you do that, you don't see much so far.
But now what I'm going to do is I'm going to make the difference even greater, OK? The first and third are very close horizontally. And the second and fourth are very close vertically. If you do that, you readily can see that indeed the first and third rows move horizontally and the second and fourth rows move vertically. So proximity is a very important factor.
And so that then brings me to an interesting question that probably none of you have given much thought. But I will make you more aware of it. The fact is that when you see movies, you very often see a car or a chariot or something move forward, but the wheels are going backwards.
And you certainly do that when you watch those endless ads on television about cars. And you see the wheels going backwards most of the time. And the car is going forward. And some of you say, well, why would I buy a car whose wheels are going backwards?
So at any rate, let's examine that question. And what I'm going to do first is I'm going to show you a movie. Ready? This a famous movie, Ben-Hur. You've probably seen it. Look at the wheels going backwards. Can you see that? Now, of course since I have given this a lot of thought, it drives me nuts when I see wheels going backwards when the chariot or the car goes forward.
All right, so now the question is, why do we see wheels going backwards in the movies or on the ads on television, whereas when you see it in the real, you never see them going backwards? Well, remember that when you look at a movie or television, you always see apparent motion. You never see real motion on television, never see real motion in the movies. You're not aware of it, but you don't. You only see apparent motion because you have successive frames in each of which there is a specific, say, if it's wheels, rotation, OK?
So let's look at that analyze this. So I'm going to show you a wheel that is rotating slowly. Are you ready? it's a very brief. OK, you can see that wheel is going forward, right? Now I'm going to show you the same and arrangement. But I'm going to have it move fast. Ready? Ready, go. That time you saw it go backwards, right?
OK, so let's analyze this. What happens when you have slow motion? Let's imagine that the red line is designating one of the spokes. Then if it moves slowly, the next frame is going to show that displaced only a little bit from the red, and so on. And because of that, you're going to see forward motion.
But now if what you do instead is moving fast, what happens is that this spoke here is going to be closer to this one in the next frame. And because of that-- of course, that's all of them all the way around-- you will see them go backwards, because of the principle of proximity that I showed you with the apparent motion of the four moving dots.
OK, so that then explains to you why you see wheels rotating backwards in the movies and on television. And it's due to a fact of the proximity of successive elements, in this case, spokes that are rotating as the picture is taken with a camera. All right.
So now I'm going to come up and tell you briefly about another very interesting phenomenon that is closely related to motion. When two successive stimuli are presented, there can be an interaction between them, all right? And in a famous series of experiments back in the 1930s, they did what is called a metacontrast experiment.
First, a disc was presented like this. And then it was followed by a ring. And they shared the same contour, OK? This is it, OK? So now what you could do-- you could vary the temporal interval between them. So if you present these two simultaneously, since they have overlapping contours, that's all you would see.
But then if you present them sequentially-- ready? Like that, then the question is, to what degree did you see that first one? So let's look at that. And I want you to pay close attention to when I present this to you, OK? Are you ready?
You're going to do it now so that they're only 60 milliseconds apart, OK? Here we go. You barely see the first one, right? You could just barely make it out. But it's not gone. Some people thought it would cause it to be gone. But that's not the case.
So what you do then is the following. If you fixate on the red cross, I'm going to present the stimuli. And I want you to tell me which of the two stimuli I present is preceded by the disc. Ready? Could you tell? Which one, left or right? Left, good. Again, repeat.
AUDIENCE: Right.
PROFESSOR: Oh. Ready?
AUDIENCE: Left. Left.
PROFESSOR: OK, very good. So the fact is that when you present two side by side where one of them is preceded by the disk, you can tell which one came first. So somehow this didn't obliterate your ability to see it. Now, the other interesting fact is that if now you flicker back and forth between them, at equal rates, you see both equally. So therefore, what happened is you did not truly obliterate the percept. You just somehow weren't too much aware of it because of the temporal offset between the successive presentations.
Now, you can do this slightly differently. Instead of having two stimuli that do not overlap but have adjacent contours, we can overlap them. So here I'm going to show you something and ask you what you see. Ready? What do you see? A big disk? OK? There it is. Now I show it to you.
As to what I really showed you, was first I presented a dim small disc followed by a brighter higher contrast bigger disc. And that obliterated the perception of the first one. And that is truly obliterated. And the interesting experiment you can do in this regard-- you can do this interocularly.
In other words, you can present one of the stimuli to the left eye and the other to the right eye. If you do that, you don't get a masking effect. But if you go back and do the metacontrast, you still get the same pseudo blockage without actually eliminating it, but sort of making you less aware of the first stimulus.
OK, so now if one examines this, the way to do the experiment right is you vary the temporal offset between the two stimuli. And when this was done systematically with the brightness masking that I showed you later, secondly, what happens is that the effect rapidly declines. It's the biggest when the two are just right next to each other.
By contrast, when you do the metacontrast, the biggest effect is when they're about 62 to 100 milliseconds separated, because that's when apparent motion is most effective. And then if you do an experiment to see where this happens for the brightness masking, here's what happens in the retina recording from a cell. This is a dim stimulus, a smaller response, and with a longer latency.
And the mask alone will get a much shorter response and a much more vigorous one. And then when you systematically vary the temporal interval between the two of them, you can see when the interval becomes short. The second stimulus obliterates the first one because it's faster and it has a bigger response.
So this explains what happens, because it happens in the retina by virtue of transmission. And the rule of the fact that the rate at which information is transmitted from the four receptors to the retinal ganglion cells is contrast dependent. The higher the contrast, the faster the response.
All right, so now does anybody have any questions about the masking or the metacontrast that we talked about so far? All right, in that case, we are now going to move on and talk about another important motion perception effect which links one to eye movement generation. And that falls into the category of so-called optokinetic nystagmus.
So how do you study optokinetic nystagmus at the very basic level? Well, the way you study it is, for example, in the olden days, you could take a large lamp shade like that and put vertical bars on int on the inside, have a person have his head inside. And then you rotate the lamp shape so that the bars go across like that. Or you can do that more simply nowadays. You just go put a bunch of vertical bars on a monitor and have them drift. And the person is asked to look at it.
So when the person does that, what you get is the so-called optokinetic nystagmus. It has two phases. It has a fast and a slow phase. The fast phase is a saccade, a resetting saccade, if you will. So you watch an edge. You follow it. And then once it gets to the periphery, you make a saccade back and you watch the next one. And you keep doing it. And this shows you the three different rates.
Now, this is quite an interesting mechanism. And it was determined that an important contribution to executing these kinds of eye movements, which is very important, because when you track anything, if you track a bird of something, you keep your eye on it. And then once it gets past you, make this a saccade back and take the next bird and track it.
So that's the mechanism. And it's been shown that several neural mechanisms play a role in it. And one of those belongs to the so-called accessory optic system, which has those cells of Dogiel in the retina that connect to it. So we're going to look at that. Some beautiful work has been done about that.
But before I do that, let me show you a very interesting factor. Here is a typical optokinetic nystagmus induced by a drifting vertical set of bars at various rates, as you can see here. And this is a rabbit. And in the rabbit, what you have is a left eye and a right eye. And you can activate them separately, as shown here.
Now, a remarkable discovery that was made is that the accessory optic system-- that there's a primary role in generating this optokinetic nystagmus that is found initially in among the cells of Dogiel, of which in the rabbit, as I've said, there are about 7,000 out of 350,000 in the eye. And it was found-- which was incredibly surprising-- that this system originating with the cells of Dogiel, the cells of Dogiel themselves get input only from ON bipolar cells, OK?
So what can we do to prove that this system here indeed is one that is activated by the cells of Dogiel, since the cells of Dogiel in the rabbit get input only from the ON bipolar cells? Anybody can think of an experiment? What did I tell you about the on and off systems and how they can be manipulated? Anybody remember?
Now, see you've got to remember facts. And one of the facts was I told you about what happens when you use 2-amino-4-phosphonobutyric acid, APB. Anybody remember that? If not, you'd better study it, because there are going to be questions on that on the exam, of course.
OK, so if you put APB into the eye, you block the ON bipolar cells. And therefore, if only ON bipolar cells feed into the cells of Dogiel, you would expect that if you block those bipolar cells, you should eliminate the optokinetic nystagmus in the eye that had been injected, if it's indeed true that the cells of Dogiel are involved, OK?
So that's a beautiful experiment, because it's a definite one if indeed the data come out the way one had hoped. And guess what happens? Here are the data. The red ones are from the eye that had been injected with APB. You know, you blocked the ON cells. And hence, you blocked the responses of the cells of Dogiel, all right?
So this is a rather nice, luckily definitive, experiment. Now, the curious thing is, if you did the same experiment in a monkey, you would get nothing. Here you do the same experiment in a monkey. Here is your OKN. And here is when you put in APB. And still after putting in APB, the monkey still does optokinetic nystagmus.
That's because the rabbit is a very unusual species. And god only knows why it happened that only On bipolar cells fed into the cells of Dogiel. In the monkey, you get both ON and OFF cells feeding into the cells of Dogiel.
All right, so now another interesting factor that I want to mention just briefly is that it's indeed is true that the feedback mechanism is essential for you to do optokinetic nystagmus right. Here is a monkey in which one eye has been immobilized and the other eye is normal. So if you look at the normal eye and you move a set of vertical bars very slowly, this is what your optokinetic nystagmus looks like-- just a very slow tracking.
But then if you do the same thing in the mobilized eye, and you, of course, record the eye movement of the other eye which is blocked, what you get is a tremendously rapid increase in the pursuit movement. This can be called open loop. And this can be called closed loop.
Now, the mechanism involved here is that your intent is to track. But when you realize-- or not you realize, but the brain realizes. You're not even aware of it-- that you are not following fast enough to keep up with the motion of the vertical bar, you order the eyes to move faster, faster, and faster, and faster and faster, and it practically runs away like crazy. And it still-- of course, because the eye is immobilized-- hasn't been able to catch up to the moving bar.
So this further highlights the importance of this mechanism. And it has to be a closed loop system, obviously, for it to work properly. So there's a feedback telling you whether you had correctly tracked something. OK, so now I come to the last point today, which is now the mechanism that is involved in the motion analysis of accessory optic system. I'll talk about this only briefly, because this is going to be part of your paper.
All right, now, they remarkable discovery that was made-- amazing discovery-- was that when people recorded from the cells of Dogiel, they found that these cells come in three different types with three different axes of orientation, these three axes. And when that was first found, people said, what on earth is going on? Why would we have three like that? I would have thought we'd have four, vertical and horizontal at least, or something.
So this was really puzzling. But then they began to do some more experiments. And they made a truly remarkable discovery. And their discovery is shown here in a summary diagram of the accessory optic system. OK, here are these cells of Dogiel with the three axes of direction selectivity. They respond to rather slow motion velocities.
So what happens here-- I'm not going to go into detail, because you're going to, as I say, report on this. But let me tell you about the essence of it. The cells of Dogiel project into the so-called terminal nuclei. And then they project up to the cerebellum. And from the cerebellum, there's a projection down to the vestibular nuclei.
And then it was found, as it was known already, that the semicircular canals come in three orientations which are the same as those of the orientations of the cells of Dogiel. And so then they feed into the ocular motor system to move the eyes so as to keep the eyes in accurate contact with whatever you're intending to look at. So that is the very essence of the accessory optic system. And I just thought this will help you in putting your paper together.
All right, so now I am ready to summarize for you. First of all, motion has been classified into several different types that include planar, circular, and radial. And one thing we talked about earlier that I mentioned here, but I didn't mention in this presentation-- when we talked about depth perception, we talked about motion parallax. That's a very important aspect of motion analysis.
Then secondly, the majority of cells in V1 and most cells in MT are directionally selective, OK? And an addition which I mentioned earlier is that they also show velocity selectivity. Some V1 cells respond to different directions of movement for light and dark edges, like the S2 type cell I showed you. And some cells are sensitive to differential velocities of movement, which I didn't show.
Then the accessory optic system that begins with the retinal ganglion cells of the cells of Dogiel form three axes of direction selectivity that correspond to the three axes of the semicircular canals. And they are involved in generating pursuit eye movements for image stabilization. Then one of the most important tasks of motion analysis is motion parallax, as it provides information about depth. Motion cues can provide important information for object recognition, often referred to as structure for motion.
And stationary stimuli that flicker with various temporary signals induce apparent motion, of which I showed you a lot of examples. And then we talked about metacontrast masking, which is a phenomenon that occurs when two stimuli share contours. And when they're presented with an interval which corresponds to apparent motion, you tend not to see the first one.
Lastly, brightness masking arises with overlapping stimuli appearing in rapid succession. And this does not occur with interocular presentation, proving that it's a record of phenomenon. And it has to do with the fact that the velocity with which a stimulus activates the retinal ganglion cells and hence, of course, the cortex, is much much slower with dim stimuli then with bright ones. And so bright stimulus, in a sense overtakes the responses to a dim and weak stimulus when the two successive presentations are very rapid.
OK, so that's the end of it here, OK? And I'll show you one more motion effect. Ready? All right, so that is the story for today. And next time, you're going to have, as I said, a rapid summary of what we have covered in the first half of this course. And then the following Wednesday, we are going to have the multiple choice exam for the midterms. OK, thank you kindly.